Defending our last line
10 Sep 2019
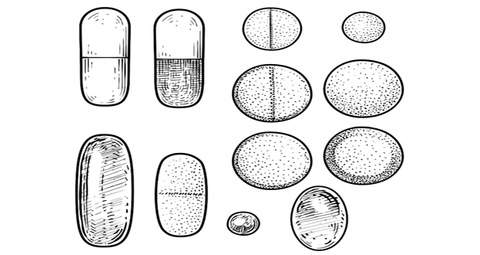
Swift action is vital in the battle against AMR. But as our last lines of defense begin to fall to a worryingly diverse number of bacterial resistance mechanisms, forget therapeutics, the real challenge is in the diagnostics…
The clinical treatment and management of infectious diseases changed significantly after the discovery of antimicrobials in the 1920s.
Of the hundreds of antibiotics developed in the intervening years, the β-lactam antibiotics are among the most important. This family of antibiotics includes the penicillins, cephalosporins, cephamycins, monobactams, and carbapenems, with the carbapenems often used as the last therapeutic option for life-threatening infections with gram-negative bacteria.1,2
However, the antibacterial activity of the carbapenems is variable. Imipenem and doripenem are effective against most gram-positive bacteria. Meropenem, ertapenem, and doripenem are effective against most gram-negative organisms. However, Enterococcus faecium is typically resistant to all carbapenems and ertapenem is not effective against Acinetobacter baumannii and Pseudomonas aeruginosa.4 Additionally, resistance to the carbapenems and to most other antibiotics is becoming increasingly common in gram-negative bacteria.3, 13
Screening patients for carriage of carbapenem-resistant organisms is important for control of infections in hospitals
The β-lactam antibiotics interfere with bacterial replication by binding to the enzymes (called penicillin binding proteins or PBPs) responsible for assembly of the bacterial cell wall.2 Bacterial resistance to the β-lactams can occur by three general mechanisms: reduced access of the antibiotic to the target, reduced binding of the antibiotic to the target, and destruction of the antibiotic.5,6
In order to reach the cell wall of gram-negative bacteria, the antibiotic must move through pores in the outer membrane. If there is a mutation in the proteins that make up the wall of these pores, then the opening may be too small to allow the antibiotic to penetrate into the cell. Additionally, there are efflux pumps in the wall of these bacteria that may simply pump the antibiotic out of the cell before it can kill the bacteria.
The second mechanism of resistance – reduced binding of the antibiotic to its target – can occur when an organism has a mutation in its PBP genes or if it acquires an altered/resistant PBP gene from another bacterium. If the protein is still able to function in building the cell wall but does not bind the antibiotic, then the bacteria become resistant to the antibiotic. The last mechanism of resistance is mediated by bacterial enzymes (i.e., β-lactamases) that break the β-lactam ring and inactivate the antibiotic. By far, this is the most common mechanism of resistance, with more than 1,300 β-lactamase enzymes identified to-date15 and grouped into one of four classes: Ambler class A, B, C, or D.
Know your β-lactamase types
Many β-lactamases that have been described are classified based on their substrate specificity. The penicillinases are enzymes that inactivate the penicillins. The cephalosporinases are enzymes that inactivate both the penicillins and cephalosporins. AmpC type cephalosporinases inactivate penicillins, cephalosporins, and cephamycins, while the extended spectrum β-lactamases (ESBLs) inactivate the monobactams as well as the penicillins and cephalosporins.
Carbapenemases inactivate virtually all β-lactam antibiotics.5 These are the ultimate β-lactamases because they potentially inactivate the whole class of antibiotics. Remarkably, even though the carbapenemases are a relatively recent addition to the antibacterial armament, many different carbapenemases have been described and are found in three of the fourβ-lactamase classes (Ambler A, B, and D).17,8
KPCs (Klebsiella pneumoniae carbapenemases) are the most common Ambler class A carbapenemases detected in Enterobacteriaceae. They are found globally and are the predominant carbapenemase found in North, Central, and South America. This carbapenemase is found in many Enterobacteriaceae, particularly in K. pneumoniae, as well as non-fermenting gram-negative bacilli including Pseudomonas. Other carbapenemases in this class include IMI, GES and SME. Ambler class B β-lactamases are called metallo-β-lactamases (MBLs) because they require zinc ions in their active sites to be able to hydrolyze β–lactams. The most common MBLs include the NDM, VIM, IMP, GIM, and SIM enzymes. NDM (New Delhi metallo-β-lactamase) was originally described in India and has now spread rapidly worldwide. VIM enzymes are also found globally and are of concern particularly in Mediterranean countries. They were first found in Pseudomonas aeruginosa but VIM-based resistance expanded into Enterobacteriaceae. At this time, the MBL class is found in many Enterobacteriaceae and non-fermenting gram-negative bacilli including Pseudomonas and Acinetobacter.
The carbapenemases in Ambler Class D are called OXA (oxacillin-hydrolyzing) and are able to hydrolyze carbapenems, some cephalosporins and penicillins; however, the hydrolysis activity of carbapenems is weak. There are several subgroups of OXA carbapenemases. A large number of them have been associated with opportunistic A. baumannii infections but they are also found in Enterobacteriaceae (predominantly in K. pneumoniae and Escherichia coli), and Pseudomonas. Of particular concern is the spread of OXA-48-containing Enterobacteriaceae. The first identified OXA-48 carbapenemase was isolated in Turkey but has spread in outbreaks throughout Europe. The majority of OXA-48 enzymes are found in Mediterranean and southern European countries, but they are also found in the Middle East and Africa.
A problem shared
Although the most common mechanism of carbapenem-resistance in gram-negative bacteria is production of a carbapenemase, an alternative mechanism also exists. Some bacteria can express carbapenem resistance by production of both a β-lactamase such as an ESBL or AmpC, combined with a porin mutation that decreases penetration of the antibiotic into the cell.6,8
This discussion of resistance mechanisms is more than an academic lesson. The genes encoding carbapenemases are frequently carried on plasmids that can be readily exchanged between bacteria.9,10 Thus, resistance can spread among patients in a hospital setting and frequently involve different strains of bacteria carrying the same resistance markers. In contrast, the alternative mechanism of carbapenem resistance is usually encoded on the bacterial chromosome and does not readily move among bacterial species. Thus, the diagnostic laboratory is confronted with multiple challenges. They need to determine if a patient is either a symptomatic or asymptomatic carrier of carbapenem-resistant organisms; if a clinical isolate is susceptible to the carbapenem antibiotics; if resistance is due to a carbapenemase and potentially at increased risk of organism-to-organism transfer; and for epidemiologic purposes, identify the specific carbapenemase.
Screening patients for carriage of carbapenem-resistant organisms is important for control of infections in hospitals.11 A patient with a recognised infection with these organisms can be appropriately isolated and treated to prevent patient-to-patient spread; however, an asymptomatic carrier can represent an unrecognised reservoir for hospital infections.12 Detection of carriers of carbapenem-resistant bacteria can be accomplished by both molecular tests and culture with selective media. Molecular tests are designed to detect the genes that code for carbapenemases. Although the tests are relatively sensitive and specific, they typically are designed to detect the most common carbapenemase genes (e.g., genes that code for KPC, NDM, IMP, VIM, OXA). Selected subtypes of these enzymes and less common carbapenemases would not be detected, as well as non-carbapenemase-producing carbapenem-resistant organisms.
In theory, culture-based methods should be able to detect all carbapenem-resistant organisms and a variety of media have been developed. This method is based on using a carbapenem to inhibit growth of susceptible organisms. However, some carbapenemase-producing organisms (CPOs) have relatively low minimum inhibitory concentrations (MICs)7 and would not be detected on these media. So the test sensitivity may vary enormously. This is also reflected in the phenotypic antibiotic susceptibility tests (ASTs). For example, a proportion of organisms producing OXA carbapenemases have MICs in the susceptible or intermediate range and would not be detected unless additional confirmatory tests are performed.7
A variety of molecular and phenotypic tests have been developed as confirmatory tests for carbapenemase production.11,13,14 The molecular tests used to test clinical isolates have the same benefits and limitations described above. The phenotypic tests can be separated into 2 groups: growth-based assays that measure carbapenem resistance based on growth in the presence of a carbapenem (e.g., modified Hodge test, modified carbapenem inactivation method [mCIM]), and tests that detect the products of carbapenems hydrolysis by carbapenemases (Carba NP).
All phenotypic tests are potentially insensitive for organisms that produce low levels of carbapenemases. For example, tests that detect the acid products of carbapenem hydrolysis measure a pH change that may be minimal if the carbapenemase is weakly active. One other limitation of these phenotypic assays is they are generally performed when the susceptibility test results indicate the organism is carbapenem-resistant. This introduces an inherent delay unless all organisms are routinely tested. One solution to this problem is the BD PhoenixTM CPO detect test. This phenotypic test is available on standard BD Phoenix AST panels, allowing for inclusion in routine susceptibility testing of Enterobacteriaceae, Pseudomonas aeruginosa and Acinetobacter baumannii.
In summary, carbapenem resistance is an evolving therapeutic and diagnostic challenge. In the last 20 years, we have witnessed the global spread of these resistant organisms, with resistance mediated by a variety of mechanisms. Resistance to the carbapenems is associated with resistance to most antibiotics so management of infected patients is challenging. Because of the diversity of the underlying mechanisms of resistance, diagnostic testing is also challenging.
Ultimately, recognition of both colonised patients and infected patients is critical for the control of infections in the hospital. If this recognition is not effective, then a century after the age of antibiotics was introduced, we could observe the end of the antibiotic era.
References:
- Bonomo R, et al. Carbapenemase-Producing organisms: a global scourge. Clin Infect Dis 2018;66:1290-1297.
- Nordmann P. et al. Global Spread of Carbapenemase-producing Enterobacteriaceae. Emerg Infect Diseases 2011 Vol. 17.No. 10Doi: 10.3201/eid1710.110655
- Oliphant M. & Eroschenko K. Antibiotic Resistance, Part 2: Gram-negative Pathogens. Journal for Nurse Practitioners 2015, Vol.1 Issue. 1. Doi: 10.1016/j.nurpa.201410.008
- Lewis , J and Bush, K. Antibacterial Agents. In: Carroll KC, Pfaller M, Landry M, McAdam A, Patel R, Richter S, Warnock D. editors. Manual of Clinical Microbiology, 12th ed. Washington, DC; American Society of Microbiology; 2019. p. 1201-1241.
- Bradford P and Castanheira M. Mechanisms of resistance to antibacterial agents. In: Carroll KC, Pfaller M., Landry M., McAdam A., Patel R., Richter S., Warnock D. editors. Manual of Clinical Microbiology, 12th ed. Washington, DC; American Society of Microbiology; 2019. p. 1242-1276.
- Tsai Y. et al. Single or in combination antimicrobial resistance mechanisms of Klebsiella pneumoniae contribute to varied susceptibility to different carbapenems. PLoS ONE 2013; 8(11):e79640.doi:10.1371.
- Karlowsky J. et al. In Vitro activity of imipenem against carbapenemase-positive Enterobacteriaceae isolates collected by the SMART Global Surveillance program from 2008 to 2014. J Clin Microbiol 2017;55:1638-1649.
- Jacoby G. et al. Role of beta-lactamases and porins in resistance to ertapenem and other beta-lactams in Klebsiella pneumoniae. Antimicrob Agents Chemother 2004;48:3203-3206.
- Queenan A. and Bush, K. Carbapenemases: the versatile beta-lactamase. Clin Microbiol Rev 2007;20:440-458.
- Mulvey M. et al. Multiple variants of Klebsiella pneumoniae producing carbapenemase in one patient. N Engl J Med 2016;375:2408-2409.
- Hrabak J. et al. Detection of carbapenemases in Enterobacteriaceae: a challenge for diagnostic microbiological laboratories. Clin Microbiol Infect 2014;20:839-853.
- Snitkin E. et al. Tracking a hospital outbreak of carbapenem-resistant Klebsiella pneumoniae with whole-genome sequencing. Sci Transl Med 2012:4:148ra116.
- Hammoud D. et al. How to detect carbapenemase producers? A literature review of phenotypic and molecular methods. J Microbiol Methods 2014;107:106-118.
- Tamma P. and Simner P. Phenotypic detection of carbapenemase-producing organisms from clinical isolates. J Clin Microbiol 2018;56:e01140-18.
- Bush K. Proliferation and significance of clinically relevant b-lactamases. 2013. Annals of the New York Academy of Sciences, Vol.1277, Iss. 1. doi: 10.1111/nyas.12023.
Authors: Dr Courtney E. Maus and Dr Patrick R. Murray, PhD are based in the Scientific Affairs department of BD Diagnostic Systems.