A potent analytical force
13 Apr 2018 by Evoluted New Media
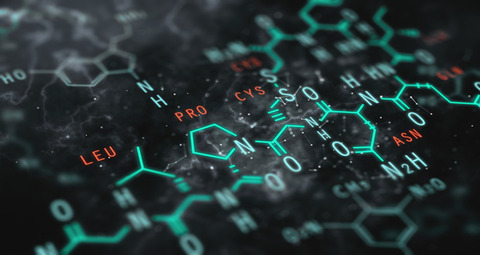
Pharma companies need more information on drug candidates at an earlier phase in the process than ever before, and analytical chemists are turning to quantitative Nuclear Magnetic Resonance (qNMR) spectrometry to give them the answers says Dr Clemens Anklin…
In today’s small molecule pharmaceutical discovery and development environment, companies must carefully balance risk and reward. In the past, analytical chemists supported the development process (pre-clinical, phase I to IV) with relevant assays at the appropriate time. Data was generated at each phase to support the ultimate submission of an NCE (new chemical entity) for regulatory approval.
With late stage clinical trials now requiring an investment of several hundred million dollars, economics dictates that minimum investment is made in a candidate until potential is proven. As a result, the development of fully validated chromatographic methods and standards – a costly and time consuming process – is often delayed. However, potency determination, traditionally done by HPLC, is critical ahead of phase I, and scientists are increasingly turning to quantitative Nuclear Magnetic Resonance (qNMR) spectrometry to provide this information.
Much has been written about how analytical chemists should best support early development. In the book Specification of Drug Substances and Products: Development and Validation of Analytical Methods, 2013, Christopher M. Riley et al, presents a comprehensive and critical analysis of the requirements and approaches to setting specifications for new pharmaceutical products, with an emphasis on phase-appropriate development and validation of analytical methods. In addition, the International Consortium on Innovation and Quality in Pharmaceutical Development, an association of more than 25 companies with a mission to advance standards and regulations for medicinal products worldwide, has published a series of guidance papers that explore and define common industry approaches and practices for applying Good Manufacturing Practice (GMP) in early development.
These, and many other reports, highlight the complex process of taking a promising candidate from discovery through the early phases of development as a drug substance (DS) and then into clinical trials as a drug product (DP). A key part of the information package needed for pre-clinical safety and toxicity testing, formulation development and first use in many studies is the accurate determination of drug potency.
Analytical options
Potency is the amount of active drug substance required to produce a given percentage of its maximum effect, irrespective of the size of the maximum effect. It has typically been measured using HPLC, however, direct measurement requires a characterised reference standard of the drug, something that most companies will not undertake for an early-stage compound. The alternative is to assess potency by subtraction, using the following calculation: Potency = Total - ? Inactive
Where the sum (?) of inactive components includes degradation substances, process impurities, water, residual solvents and inorganic material.
As part of the analytical approach to this subtraction protocol, parallel measurements of absolute compound purity assessment, identity testing, residual solvent, moisture analysis, relative response factor calculation are often performed. Naturally, scientists have looked for a technology that could offer a ‘one-stop’ option in order to simplify, automate and reduce the cost of analysis.
If not HPLC, then what alternative technologies can help – quantitative UV–Vis spectrophotometry, quantitative infrared, or mass spectrometry? Any technique must be ‘rapid’ and selective for the drug being characterised in the presence of likely impurities, and it must be quantitative without the availability of a characterised standard for the specific drug. UV–vis spectrophotometry is rapid but is not selective for impurities and needs a characterised drug standard. Infrared and mass spectrometry can be selective for likely impurities but also require a characterised standard for quantification.
A consensus has now emerged that q-NMR provided the ideal solution to this problem. Potency determination by qNMR has been shown to be a single point replacement for routine development testing which previously involved several experiments and techniques1. Importantly, NMR also provides structural information and confirms compound identity within the same experiment. From a regulatory standing, traditional and quantitative NMR is accepted by the International Conference on Harmonization (ICH).
The only downside to qNMR has been that certain workflows were considered to need expert knowledge and/or well-trained analysts and detailed protocols.
qNMR in practice
Today, new-generation qNMR instruments, workflows that use a commercial internal standard (IS), and application-specific software have made qNMR a routine experiment. In many companies, qNMR is now installed as an ‘open access’ system that all chemists can use. There are three simple steps – sample prep, sample submission and automated data analysis and reporting – required by a typical rapid and automated workflow.
Optimised qNMR experiments are usually provided as well as parameters for most commonly used internal standards. Automated data analysis often comprises internal standard peak identification, analyte quantification, consistency analysis and potency calculation. Modification of the workflow according to SOPs which define the analyte's integral regions can be made.
Error analysis is also automated within the sample, with multiple analyte peaks integrated, averaged and the RSD given. Between samples, replicate samples can be submitted. Potency for each replicate will be calculated and a final averaged result and any associated error reported.
NMR has long been used in quantitative applications, it is, after all, an inherently quantitative technique. But only with the availability of new systems and new automated workflows, has qNMR become the method of choice for early potency determination in small molecule development groups. Interestingly, qNMR has emerged at a time when regulatory bodies require companies to detailed knowledge of a candidate drug early in the development process, and economic pressures are driving changes from the established ways of doing things. The technique is already making a difference, and looks set to become even more widely applied.
[box type="shadow" align="aligncenter" ]NMR basics NMR relies on a property of certain atomic nuclei that causes them to absorb, then re-release, electromagnetic energy at characteristic frequencies. Shifts in the usual response frequency for a given isotope provide information about their immediate environment, including influences from nearby electrons and magnetic nuclei, making it possible to infer molecular identity, geometry, and more. Importantly, the NMR signal is also directly proportional to the number of spins present in the sample. Thus making NMR a primary ratio quantitative method. Here is an overview of the process: Sample preparation: NMR requires in general very little sample preparation. For liquids NMR the material is dissolved in a suitable solvent. Liquid samples can even be measured in the natural state. For solid samples NMR the materials are typically packed into a small diameter cylindrical rotor. Sample introduction: A thin-walled glass tube containing the sample is placed inside an NMR probe which consists of a radio frequency coil and tuning circuitry, which in turn sits inside a powerful magnet at the heart of the NMR spectrometer. The magnet causes susceptible atomic nuclei within the sample to align with its field, giving them a consistent resting alignment. NMR specifically applies to nuclei that contain an odd number of protons and/or neutrons, for example, 1H or 13C. These nuclei exhibit a built-in magnetic moment and angular momentum that together give the nuclei a property called ‘spin’. A strong enough magnet will cause these nuclei to align their spins with its field. Data acquisition: The radio frequency coil releases one or more radio-frequency pulses at the right frequency to perturb specific nuclei, then detects the energy released as the nuclei “relax” back to their resting alignments in a process called free-induction decay (FID). Because the FID signal can be quite small relative to background noise, multiple acquisitions are often averaged. That signal is then converted by Fourier transformation into an NMR spectrum showing the frequencies at which the nuclei responded. Data interpretation: Well-characterised differences in the response frequencies for a given isotope reveal the electromagnetic influences of neighbouring electrons (“chemical shift”). Splitting of peaks into sub-peaks indicate magnetic influences of neighbouring nuclei (“spin coupling”) giving information about number and geometry of these. The NMR signal is also directly proportional to the number of spins present in the sample. Thus making NMR a primary ratio quantitative method. Customised sequences of RF pulses tease out specific details about a sample, sometimes probing multiple nuclei. Advanced software can simplify analysis and interpretation, and automate many aspects of data acquisition, analysis, and reporting. [/box]
References
- Webster G.F. and Kumar S., Anal Chem, 86, 11474 (2014)
Author:
Dr Clemens Anklin, is VP at Bruker BioSpin Corp. He collected his first NMR spectra in 1978 while being a student at ETH In Zurich, Switzerland. He started work at Bruker in 1984, in Switzerland, as an application scientist.