The legacy of Hawking radiation
14 Mar 2018 by Evoluted New Media
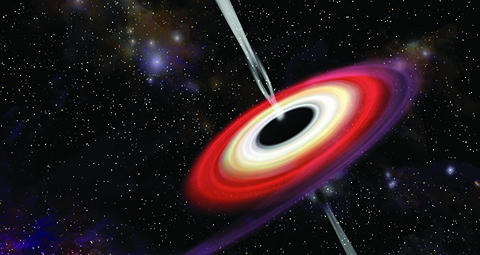
Perhaps the most powerful legacy of Stephen Hawking was the inspiration he gave to others – but his science on black holes will endure...
Perhaps the most powerful legacy of Stephen Hawking was the inspiration he gave to others – but his science on black holes will endure. After a 40 year wrestle with the laws of physics, has the time come to claim the information paradox associated with black holes is over? By accepting a neat quirk of quantum mechanics Professor Christoph Adami thinks so…
Black holes are awesome. They are mysterious and dark and very heavy. We have a supergiant black hole right smack in the middle of our own Milky Way that weighs in at about 4 million times the mass of our sun. There’s even one not that far away from us, a mere six thousand light years away – practically our neighbour. Named Cygnus X-1 – is only about 15 times as heavy as our sun, so not quite as voracious as Sagittarius A*, the black hole around which our whole galaxy revolves.
Black holes are black because they are so heavy that even light can’t escape their gravitational pull. However, the feature that has thrown the theoretical physics community into a tizzy for the last 40 years isn’t their blackness. It is a very peculiar feature of black holes that they are characterised only by their mass (if they aren’t rotating or charged, that is). To see that such a fact may present a problem to the integrity of all of physics takes a little bit of thinking, but it is worth the effort. Suppose I throw two books into a black hole. Let us say that both books weigh the exact same amount, but let their text be utterly different. I like throwing in Darwin’s Origin of Species and Shakespeare’s Hamlet, (the Hamlet must be the pocket edition, as it is less than a fifth in length compared to Origin of Species). Whichever is tossed, after the tome is swallowed by the black hole the Universe looks exactly the same way. The mass of the black hole has increased, but whether it is the Prince of Denmark that led to this inflation or Darwin’s trepidations which caused the swell can’t be known. That information is lost, and herein lays the problem.
The laws of physics have a very peculiar feature, namely that everything that happens can also happen in reverse. This law, called “time-reversal invariance” obviously does not hold for large things: shattering a glass on the floor will happen only in one time direction: forward. But the law does hold for atomic particles, and experiments have upheld this law time and time again. The reason why the law does not hold for aggregates such as water melons is entirely statistical, by the way: it is because on average, disorder increases. But what holds for the tomes of Darwin or the bard must hold for particles too, and that is why black holes are feared by the theoretical physics community: they threaten our most closely held truths. Indeed, how could you reverse time if the identity of the book you threw into the black hole was lost? It would be impossible.
43 years ago, Stephen Hawking announced that back holes aren’t so black after all, and that they do emit a “glow” that is now called Hawking radiation
43 years ago, the theoretical physicist Stephen Hawking announced that back holes aren’t so black after all, and that they do emit a “glow” that is now called Hawking radiation¹. This effect cannot be understood using our notions of classical physics, but instead is based on the quantum laws of physics that govern all sub-atomic interactions. With this discovery came the hope that the information loss problem would fall to the wayside too, but instead it appeared to make matters worse. Hawking showed that the stuff coming out of a black hole must be random, there cannot be any information in it. With that, the information paradox became even more acute – now the loss of information was cemented into quantum physics.
Many a researcher in the field of quantum gravity in the last 40 years has wandered the wilderness of lost information in vain. ‘The Promised Land’, namely the unification of the laws of gravity and the laws of quantum mechanics has remained elusive. Will we have to abandon our hopes for a consistent description of happenings at the event horizon? New evidence has sprouted in the desert of ideas that points toward a simple and elegant solution to the troublesome question – one that carries the fingerprints of one of theoretical physics’ most revered deities; Albert Einstein.
Einstein studied black bodies in 1917, but they were not black holes. Instead, Einstein tried to reconcile the laws of quantum mechanics that he helped discover with the laws that govern the interaction of matter and light. When light interacts with matter, Einstein found that three things happen². One – light is absorbed (hence the blackness). Two – matter also glows all on its own, depending on the temperature of the body (this is precisely the effect that Hawking had discovered some 60 years later in the context of astrophysics). Three – matter emits radiation in response to what is absorbed by the black body. The discovery of this form of radiation, called “stimulated emission” (because it is stimulated by the radiation incident on the black body) revolutionised physics, because it is the effect that makes Lasers possible (the “ser” in Laser stand for “stimulated emission radiation”).
Now, it is not immediately clear that black holes are also black bodies, and that they therefore must conform to the three rules of Einstein. But work performed shortly after Hawking’s discovery – as well as work that I have pursued over the last ten years – suggests so. My own take on the problem is influenced by more modern tools of theoretical physics that have allowed us to quantify information mathematically, and in particular clarified the concept of information in the realm of quantum physics.
Couched in the language of the quantum communication specialist, the age-old problem of information loss can be re-cast as asking whether it is possible to transmit information over a channel that has a black hole smack in the middle. The classical theory of information, codified by the eminent mathematician and engineer Claude Shannon in 1948, gave us the formulas that allow us to calculate how much information can be sent through a channel with arbitrary accuracy. Hawking’s calculation from 1975, when viewed from this angle, gives the result zero, even when the modern quantum-theoretic extensions of Shannon’s formulas are used. A vanishing communication capacity means trouble for physics.
However, Hawking had disregarded Einstein’s stimulated emission in his calculations. And even though well-known physicists had pointed out this shortcoming in the years following Hawking’s announcement 3,4 the crucial role of stimulated emission in solving the information paradox would not be appreciated until recently.
To appreciate the importance of stimulated emission, we have to take a step back to understand where it comes from. Both of the forms of radiation that Einstein discussed – the spontaneous form and the stimulated kind – are profoundly quantum in nature. As you may know, the quantum world is not the world we are used to in everyday life. If you could shrink yourself to atomic size (and, honestly, quite a bit smaller), you would be amazed. Things can appear out of nothing (pairs of things actually, particles and their anti-particles), and just after they appear, they annihilate in a flash. This happens most often for light particles (light here meaning “not heavy”), but once in a while even a beast of a particle and their mirror image appear – and then disappear again. This phenomenon is called “spontaneous emission” – it is space-time “burping”, if you wish. This is also what makes Hawking radiation possible: imagine space-time burps a particle-anti-particle pair just at the event horizon, and one of the two is swallowed. The other, because it was moving in the opposite direction, escapes and thus never annihilates. It just moves outward – and makes the black hole look like it’s glowing.
Happy 40th birthday Hawking radiation. Your long roundabout journey has come to an end – you do not violate any of the laws we hold so dear
But empty space can also be tickled. If, under the right circumstances, you throw a particle (the tickler) at the right material – that material can respond by creating a pair. A particle-anti-particle pair just like those that form spontaneously, except this pair tries to imitate the tickler.
It’s really a copy and an anti-copy of the tickler particle (you always have to produce things in pairs). This is stimulated emission: the process Einstein discovered and that makes the Laser possible. Imagine that you put a mirror there so that the copy is reflected and becomes the tickler. That stimulates another copy, which becomes the tickler and so on. Soon enough you have a cascade of identical particles (in this case photons – the particles of light), i.e. a Laser.
It so happens that black holes react just like those lasing materials, as several people have shown. And it is precisely this effect that ends the 40 years of wondering where the information in a black hole went. It never really disappeared, because just before any particle crosses the line of no return (the event horizon), it stimulates the black hole to burp a copy of it. It is nature’s way of an insurance policy (because information should not ever disappear) so as to ensure the integrity of our future – the black hole is, yours, mine, the entire Universe.
40 years is a long time to walk in the wilderness. Why did it take so long to figure out where the information is? I think many factors are to blame. First, Hawking dismissed stimulated emission right off the bat in his paper 40 years ago. While several authors noticed the omission, they did not think that it would solve the problem. This is, I think, because the mathematical theory describing information transmission in the quantum world did not exist at the time: it emerged in the early 90s. The question: “Can you communicate through a quantum black hole?” could not have been answered before that time. But finally it has, and the answer is “Yes, yes you can!”5,6,7. And that means that physics survives another challenge, as it always does. Happy 40th birthday Hawking radiation. Your long roundabout journey has come to an end – you do not violate any of the laws we hold so dear.
References:
1. S. W. Hawking, Particle creation by black holes. Commun. Math. Phys. 43 (1975) 199-220. 2. A. Einstein, Zur Quantentheorie der Strahlung. Physik. Zeitschr.18 (1917) 121--128. 3. R.M. Wald. Stimulated-emission effects in particle creation near black holes Phys. Rev. D15 (1976) 3176–82. 4. J.D. Bekenstein and A. Meisels. Einstein A and B coefficients for a black hole Phys. Rev. D15 (1977) 2775. 5. C. Adami and G. Ver Steeg, Classical information transmission capacity of quantum black holes. Class. Quantum Grav. 31 (2014) 075015. 6. K. Bradler and C. Adami. The capacity of black holes to transmit quantum information. J. High Energy Phys. JHEP05 (2014) 095. 7. C. Adami and G. Ver Steeg. Black holes are almost optimal quantum cloners. J. Phys. A: Math. Theor. 48 (2015) 23FT01.
The author:
Christoph Adami is a professor of Physics and Astronomy, as well as professor of Microbiology and Molecular Genetics, at Michigan State University. He obtained his PhD and M.A. in theoretical physics from the State University of New York at Stony Brook, as well as a Diploma in Physics from Bonn University.