An instrument-free future?
16 Feb 2017 by Evoluted New Media
Molecular diagnostics requires dedicated equipment and electricity – this can present a barrier to access to those without sufficient resources. But what if we could visually analyse diagnostic tests without instrumentation?
Molecular diagnostics requires dedicated equipment and electricity – this can present a barrier to access to those without sufficient resources. But what if we could visually analyse diagnostic tests without instrumentation? Nathan Tanner presents a “sans technology” future…
The relative ease of discovery, and sheer amount, of DNA sequence knowledge available is benefiting modern life sciences by providing new information about the genetic makeup of life. As we discover new genetic aspects of human health and its threats, the central dogma of molecular biology still holds: all organisms and viruses are a result of their genetic code. And fortunately for humanity, science has gotten pretty good at reading and using this code.
Methods looking for a particular sequence for a health or detection purpose fall into the category of “molecular diagnostics”
Nucleic acids, both DNA and RNA, are routinely analysed, sequenced and manipulated, and with the ability to know the precise arrangements of their nucleobases, any specimen of interest accordingly carries a unique genetic identifier. The tools of modern molecular biology, namely nucleic acid extraction and amplification techniques, enable the scientist to analyse a sample for the presence or absence of any genetic sequence. Methods looking for a particular sequence for a health or detection purpose fall into the category of “molecular diagnostics”. Applications of these methods are widespread, from a laboratory scientist analysing the expression level of a gene of interest to a blood-testing facility monitoring the safety of the blood supply. Recent developments are lowering the barriers to entry for nucleic acid testing, with a rise in the simplicity of the tests matching that of the accessibility of the sequence information.
This mainstreaming began in the 1980s, as the polymerase chain reaction (PCR) combined two key developments: the ability to readily create short, specific pieces of DNA (oligonucleotides) and use of these pieces as “primers” to direct the activity of a DNA polymerase that could survive the high temperatures used to melt double-stranded DNA. Rounds of melting, priming and extension result in an exponential increase in the amount of a specific piece of DNA in a reaction, and that product could be used for molecular cloning or be analysed in myriad ways once made at sufficient scale. PCR has grown dramatically since its introduction, and it remains the core diagnostic method for nucleic acid amplification tests (NAAT). PCR is used for gene assembly, quantitation with real-time detection (qPCR), and rapid cycling to enable diagnostic assays. However, all PCR methods still require an instrument capable of quick heating and cooling between 95°C and about 60°C, along with some method to analyse the product: either a detection during amplification using real-time fluorimetry, or a post-reaction analysis using manipulation like gel electrophoresis.A significant focus of diagnostics development has been to further the use of “point-of-care” or “point-of-need” testing, which is to have the actual diagnostic assay at the location where a sample is collected or a patient is treated. This on-demand testing obviates some of the biggest hurdles to wider use of diagnostic tests and their follow-on treatments. In other words, it can be difficult to ensure the bidirectional flow of information accompanying a laboratory test. As an example, imagine that a patient in Ghana is tested for onchocerciasis (river blindness). A sample may be collected from the patient but must be transported back to a laboratory, evaluated for the parasite and, importantly, the result must be communicated back to the patient and treatment issued, in this case with ivermectin. Additional tests for the presence of a second parasite, Loa loa, may also be required. This requires a clarity and maintenance of information about the sample, actual transport and handling of the sample, and the ability to locate the patient once the result is determined. All these potential problems could have been eliminated if the test were performed on site and treatment and follow-up issued immediately.
The key feature underlying all isothermal methods (and there are many) is strand displacement, or the ability of some DNA polymerases to synthesise DNA without a denaturation step
Physicians, farmers and food manufacturers all benefit from the ability to perform rapid, simple tests at the location of sample collection. However, the typical demands of a PCR assay require equipment and infrastructure that are difficult to move around with the user. The use of isothermal, or single-temperature amplification techniques can help overcome these demands. The key feature underlying all isothermal methods (and there are many) is strand displacement, or the ability of some DNA polymerases to synthesise DNA without a denaturation step. This allows for an isothermal reaction to be carried out at one temperature, and for the rate of the reaction to be limited only by the enzyme and the copy number of the target rather than strictly set by temperature cycling, enabling very fast reactions.
One popular isothermal technique, Loop Mediated Isothermal Amplification (LAMP), was developed using one of the first commercial strand-displacing polymerases, the large fragment of Bacillus stearothermophilus DNA Polymerase I, and unique primers that form the “loop” structures of the name to facilitate rapid and extensive synthesis¹,². The large amounts of DNA made in LAMP reactions lead to rapid detection of product when coupled with fluorescent indicators, and early reports even validated visual detection of amplification by use of metal binding indicators such as calcein³ and hydroxynaphthol blue?. LAMP has become a widely used molecular diagnostic method, with FDA-cleared assays on Meridian’s illumigene platform (joining nicking enzyme amplification reaction assays from Alere, helicase dependent amplification assays from Quidel and transcription mediated amplification assays from Hologic as cleared isothermal diagnostics). The breadth of LAMP assays is astonishing, with 2000 publications to date in fields ranging from infectious disease, food safety, and clinical diagnostics to agricultural testing and livestock breeding. Many of these reports will use or suggest a visual indication of amplification: turbidity through precipitation of magnesium pyrophosphate?, or a metal indicator.[caption id="attachment_57977" align="alignnone" width="620"] With only a low-cost heat source and your eyes, colorimetric LAMP has many potentially powerful uses, including easy and rapid detection of infectious disease like river blindness.[/caption]
As a reagent provider, New England Biolabs (NEB) has long developed and studied enzymes for molecular biology applications, and has a strong interest in polymerases for isothermal amplification and providing reagents to many of the researchers developing these assays. Feedback from customers indicated that readouts such as visual turbidity/precipitation or the metal binding dyes did not quite work consistently enough in practice. NEB has significant research efforts devoted to LAMP and the development of isothermal polymerases and methods. Data collected as part of these efforts indicated that while real-time turbidity or UV illumination of calcein worked well, using them for a visual indicator was not sufficiently clear or easy to use for point-of-care applications and testing in the field. One experimental condition NEB was curious about was how well its reactions and polymerases would perform when tested in a very low buffer environment. Buffers (e.g. Tris, Phosphate, HEPES, etc.) are used in effectively every molecular biology reaction. However, one specific aspect of DNA polymerase activity made this experiment particularly interesting: with every incorporation of a deoxynucleoside triphosphate into the growing new DNA strand, the polymerase also produces a pyrophosphate group and a proton. The pyrophosphate can be coupled to a detection mechanism, but the proton is typically ignored as the reactions are sufficiently buffered by the reaction solution. What would happen in a LAMP reaction without any buffer?
Returning to the example of river blindness, an NEB parasitology research group demonstrated the use of the colorimetric LAMP method directly with skin snips for easy and rapid detection of onchocerciasis
LAMP can make an extraordinary amount of DNA (up to 50x that of a PCR reaction²,?), so a lot of dNTPs are consumed and accordingly a large number of protons released during the course of a LAMP reaction. Therefore we prepared LAMP reactions with all the components in a standard assay, but without Tris buffer, adjusted the pH to approximately pH 8.8, and let it incubate for an hour. Following the reaction, the pH was measured again, and it had dropped by an impressive 2.5 units?. A pH shift of this size is easily measured by pH paper or other indicators, and by simply including in the mixture a visible pH-sensitive dye, we could produce a clear, visual detection of amplification in 30 minutes or less?. This principle was further developed into a stable formulation using a pink to yellow transition (NEB’s WarmStart Colorimetric LAMP 2X Master Mix (DNA & RNA), NEB #M1800), which is commercially available and provided to a wide range of diagnostic assay developers to enable better field-friendly, point-of-care tests. Returning to the example of river blindness, an NEB parasitology research group demonstrated the use of the colorimetric LAMP method directly with skin snips for easy and rapid detection of onchocerciasis?. Tests like this extend the reach of the diagnostics laboratory to the field with simpler and more rapid tests, which allows for better on-site testing and treatment.
Many other methods are extending their field reach as well, with portable and/or battery-operated instruments available for isothermal amplification from Qiagen, Douglas Scientific, and Optigene; no-power solutions such as the NINA thermos heater from PATH; and simple PCR instruments from Amplyus (miniPCR), Tetracore, Ubiquitome and others. These instruments provide a huge step towards democratising diagnostics and offering access to previously cost- and expertise-prohibitive molecular biology tools.
The wide availability of sequence information and stable reagents, combined with the affordability of oligonucleotide synthesis, are empowering life science research and testing around the world. Efforts like NEB’s colorimetric approach to LAMP and others can even remove the need for an instrument altogether, with warm water and eyes being all one needs for a robust diagnostic assay. DNA and RNA extraction methods continue to improve in efficiency and simplicity to allow easy use of blood or other biological samples. And as the tolerance and performance of assays like colorimetric LAMP grows, users will be able to directly test a patient sample?,?, plant¹? or animal material¹¹, or insect¹² in the reaction. A truly instrument-free approach will have a broad impact on where science can be done and extend the reach and power of the genomic age to serve human health worldwide.
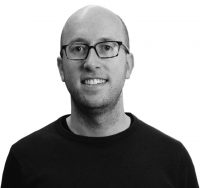
References 1. Notomi, T. et al (2000). Loop-mediated isothermal amplification of DNA. Nucleic Acids Res. 28(12):e63. 2. Nagamine, K., Hase, T., and Notomi T. (2002). Accelerated reaction by loop-mediated isothermal amplification using loop primers. Mol Cell Probes 16(3):223-9. 3. Tomita, N., Mori, Y., Kanda, H., and Notomi, T. (2008). Loop-mediated isothermal amplification (LAMP) of gene sequences and simple visual detection of products. Nat Protoc. 3(5):877-82. 4. Goto, M., Honda, E., Ogura, A., Nomoto, A., Hanaki, K. (2009). Colorimetric detection of loop-mediated isothermal amplification reaction by using hydroxy naphthol blue. Biotechniques 46:167-72. 5. Mori., Y., Nagamine, K., Tomita, N., and Notomi, T. (2001). Detection of loop-mediated isothermal amplification reaction by turbidity derived from magnesium pyrophosphate formation. Biochem Biophys Res Comm. 289:150-4. 6. Tanner, N.A., Zhang, Y., and Evans, T.C. Jr. (2015). Visual detection of isothermal nucleic acid amplification using pH-sensitive dyes. Biotechniques 58(2):59-68. 7. Alhassan et al. (2016). Comparison of a new visual isothermal nucleic acid amplification test with PCR and skin snip analysis for diagnosis of onchocerciasis in humans. Mol Biochem Parasitol 210(1-2):10-12. 8. Edwards, T., Burke, P.A., Smalley, H.B., Gillies, L, and Hobbs, G. (2014). Loop-mediated isothermal amplification test for detection of Neisseria gonorrhoeae in urine samples and tolerance of the assay to the presence of urea. J Clin Microbiol. 52(6):2163-5. 9. Hayashida, K., Kajino, K., Hachaambwa, L., Namangala, B., and Sugimoto, C. (2015). Direct blood dry LAMP: a rapid, stable, and easy diagnostic tool for human african trypanosomiasis. PLoS Negl Trop Dis. 9(3):e0003578. 10. Tsai, C., Shih, H., Ko, Y., Wang, R., Li, S., and Chiang, Y. (2016). Direct LAMP assay without prior DNA purification for sex determination of papaya. Int J Mol Sci. 17(10):e1630. 11. Abdulmawjood, A., Grabowski, N., Fohler, S., Kittler, S., Nagengast, H., and Klein, G. (2014). Development of loop-mediated isothermal amplification assay of rapid and sensitive identification of ostrich meat. PLoS One 9(6):e100717. 12. Alhassan, A., Makepeace, B.L., LaCourse, E.J., Osei-Atweneboana, M.Y., and Carlow, C.K. (2014). A simple isothermal DNA amplification method to screen black flies for onchocerca volvulus infection. PLoS One 9(10):e108927.