Where is the Universe’s ordinary matter?
3 Mar 2016 by Evoluted New Media
While we know how much matter was created at the Big Bang we can only observe a fraction of it. Forget dark matter – the question is: Where is all the ordinary matter?
While we know how much matter was created at the Big Bang we can only observe a fraction of it. Forget dark matter – the question is: Where is all the ordinary matter?
Shortly after the Big Bang (some 13 billion years ago), the Universe was in a hot, dense and homogeneous state. The matter density was so high that radiation was continuously absorbed and re-emitted, such that light could not travel freely across the Universe. The Universe then expanded and matter cooled down, such that 380,000 years after the Big Bang, electrons and nuclei recombined to form atoms. At this stage, light could finally escape and fill the Universe with a global radiation field. This “echo” of the Big Bang, known as the cosmic microwave background, was observed for the first time in 1964 and provides us with a wealth of information on the initial stages of the Universe. Many experiments have studied the cosmic microwave background and have been instrumental in shaping our current knowledge of cosmology. Tiny fluctuations in the temperature of the cosmic microwave background tell us about the distribution of matter in the early Universe and are very sensitive to the global matter content.
In January 2015, the results of the Planck satellite of the European Space Agency (ESA) were published. The high sensitivity of Planck allowed us to estimate the amount of matter and energy in the Universe with a precision of just a few per cent. In particular, we now know that all forms of matter as we know them (atoms, radiation, and all sorts of exotic particles) only account for 16% of the Universe’s matter, the rest being in an unknown form that is usually referred to as dark matter. While the exact form taken by dark matter is one of the greatest mysteries of today’s physics, its effects on the formation of structures in the Universe are now relatively well understood. Indeed, as the main matter component, dark matter governs the formation and evolution of cosmic structures, leading to the formation of the galaxies that we see today. From the small fluctuations in the primordial matter distribution that we observe in the cosmic microwave background, it is expected that over-dense regions will grow under the action of gravity by attracting the surrounding material, thus forming bigger and bigger structures. As a result, over time the Universe becomes more and more inhomogeneous, leading to the amazing diversity of size and shapes that we see in the galaxies around us. Galaxies organise themselves into an interconnected network of filamentary structures, the cosmic web. At the knots of the cosmic web we observe the most massive structures in today’s Universe – galaxy clusters.Galaxy clusters are gigantic structures that can span as much as 10 million light years on a side. They contain thousands of individual galaxies, each of them composed of billions of stars.
Galaxy clusters are gigantic structures that can span as much as 10 million light years on a side. They contain thousands of individual galaxies, each of them composed of billions of stars. Even though the stellar content of these structures is impressive, all the stars in the galaxies only make up a very small fraction (1-2%) of the material contained within galaxy clusters. Just like for the Universe as a whole, dark matter is the dominant component (85%) of galaxy clusters. Interestingly, the stellar component represents a small fraction of the total amount of ordinary matter as well. Large-scale galaxy surveys have shown that this conclusion can be generalised to the Universe as a whole. Therefore, although the total amount of ordinary matter that was formed during the Big Bang is very well known, in the Universe around us we are only observing a fraction of the expected quantity of ordinary matter. A census has shown that about half of the ordinary matter is still missing.
[caption id="attachment_52449" align="alignnone" width="540"] Most matter in galaxy clusters are made of very hot gases of temperatures up to 100 million degrees Celsius.[/caption]
In the early 1970s, X-ray observations revealed that most of the ordinary matter in galaxy clusters is in the form of a very hot and tenuous gas that fills their entire volume, with temperatures between 10 and 100 million degrees. Because of its extremely high temperature, this plasma emits energetic X-ray light which current space observatories (XMM-Newton, Chandra) are capable of detecting. During the formation process, the gas located in the vicinity of a massive structure is attracted gravitationally and falls within the cluster. It gains energy and gets heated to these incredibly high temperatures. Computer simulations tracing the evolution of cosmic structures have shown that this process is ubiquitous across the Universe. The majority of the ordinary matter should be in the form of a hot plasma with temperatures exceeding 100,000 degrees that fills not only galaxy clusters, but also the entire filamentary structure of the cosmic web – inside which, galaxies and galaxy clusters are embedded. The existence of this medium is a long-standing prediction of numerical models of galaxy formation. However, because of the low densities involved, this plasma emits very little X-ray light, such that it has remained undetected so far.
To this aim, we turned to Abell 2744, a very massive galaxy cluster located 4 billion light years away.
Since galaxy clusters are located at the crossroads of filaments in the cosmic web, they are favourable locations to search for hot gas associated with the cosmic web, and thus for the missing ordinary matter in the Universe. To this aim, we turned to Abell 2744, a very massive galaxy cluster located 4 billion light years away. Because of the huge mass of this particular system, we expected it to be connected to several filaments, inside which the density of the gas should be relatively high. We used a long exposure of this region using ESA’s XMM-Newton space telescope to map the distribution of hot gas in and around Abell 2744, and we detected three filamentary structures with temperatures of 10 million degrees radially connected to the main structure.
[caption id="attachment_52450" align="alignnone" width="540"] Three filamentary structures associated with hot gas were detected in Abell 2744[/caption]
We used optical images to study the distribution of the galaxies in the region, and found numerous galaxies associated with the position of the hot gas. Therefore, we have indeed discovered large amounts of hot gas associated with the filamentary structure of the cosmic web. We measured the shapes of distant galaxies to look for the gravitational lensing effect. This effect occurs when the light emitted by a distant galaxy passes close to a massive structure located along the line of sight. As predicted in Einstein’s general theory of relativity, the geometry of space-time is curved by the presence of the mass concentration, such that the light path is deflected and the image of the distant galaxy is distorted.
We found that the hot gas amounts to about 10% of the mass of the filaments, which is close to the fraction of 16% of ordinary matter contained within the Universe as a whole.
The amplitude of the gravitational lensing effect directly depends on how much mass is contained within the foreground structure. By measuring this effect, we could estimate the total amount of matter contained within the filaments, and we could compare this value with the mass of the hot gas. We found that the hot gas amounts to about 10% of the mass of the filaments, which is close to the fraction of 16% of ordinary matter contained within the Universe as a whole.
The properties of this gas are very similar to the predictions of computer simulations. Therefore, our observations provide an important confirmation of the picture in which most of the ordinary matter of the Universe is in the form of a very hot and diffuse gas that fills the large-scale dark matter structures. However, to draw such a conclusion we must verify that the filaments of Abell 2744 are not a special place in the cosmic web, and that similar properties are found throughout a representative region of the Universe. This is one of the main scientific goals of the ATHENA project, which is now being designed by ESA. ATHENA has been selected by ESA in 2014 to become the successor of XMM-Newton. The ATHENA telescope, which is scheduled for launch in the mid-2020s, will collect 10 times more X-ray light than its predecessor, which will allow the astronomers to make a complete census of the hot gas contained within the cosmic web. The properties of the hot gas will be studied in details to validate our understanding of how galaxies form in the Universe.Author
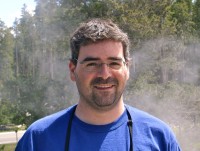
Dr Dominique Eckert is a researcher at the University of Geneva in Switzerland. He is a specialist of galaxy clusters and observational cosmology.