Swimming out from an evolutionary shadow
14 Jan 2016 by Evoluted New Media
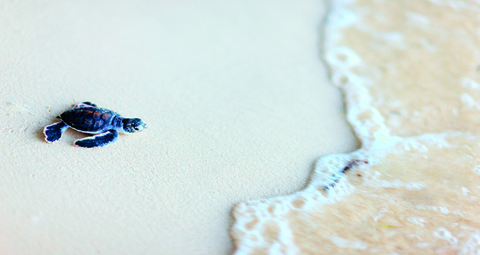
We hear how the humble turtle has been problematic to classify correctly in an evolutionary context, and how hard work and a spot of luck has changed this…
We hear how the humble turtle has been problematic to classify correctly in an evolutionary context, and how hard work and a spot of luck has changed this…
The heuristic model underlying all comparative biology is simple, elegant, and powerful. Its fundamental purpose is to identify the events populating the evolutionary space, created through extinction or sampling bias, that separates any two extant groups of organisms (a.k.a., crown clades). These transformations are not only responsible for the biologically unique nature of all crown clades, their identification reveals what features two extant lineages conserve from their common ancestor, which in many cases is even more critical to the diverse goals of biological research. The model reconstructs these histories almost exclusively through a combination of empirical observations made within the crown clades (most generally using exemplar taxa developed as model organisms for the laboratory) and justified inferences performed along those clades’ individual stem lineages. The overall success of this approach is without question, but it does have its weaknesses.The model suffers an erosion of explanatory power if significant conflict regarding the phylogenetic relationships of the compared crown clades. Also, if the phylogenetic stems separating those clades in deep time are witness to evolutionary events so large in number and scope that comparisons made between the crown clades are unable to adequately capture the nature of those events. Sometimes, however, researchers get lucky, and they are provided little empirical windows allowing them to gaze directly into the deep history of these phylogenetic stems. These windows are, of course, in the form of fossils – fossils that can be critically helpful in chopping those long evolutionary branches into smaller, more manageable pieces.
The two described sources of heuristic weakness in the standard comparative model both afflict the long puzzled-over evolutionary origin of turtles. In the last five years alone, systematic analyses have recovered turtles as: 1)basally diverging lineage of an otherwise extinct radiation of early reptiles called parareptiles; 2)deeply nested parareptiles closely related to a group of animals called the pareiasaurs; 3)away from parareptiles but still outside a crown-clade known as Sauria, which is defined by the split between the lineage that would eventually produce modern lizards and snakes (Lepidosauria) and the lineage that would eventually produce modern crocodilians and birds (Archosauria); 4) inside Sauria but more closely related to lepidosaurs; and finally 5) inside Sauria but more closely related to archosaurs.
The latter solution is nearly consistently recovered by molecular-based analyses (e.g. nucleotide sequences of mitochondrial and nuclear genes, microRNAs), but the dizzying nature of alternative morphological solutions is a testament to both the highly derived nature of the modern turtle body plan and the absence of clearly transitional fossils.
From its original description in 1887 until just recently, the Late Triassic Proganochelys quenstedtii was the best known example of an early, stem-group turtle. Approximately 50 million years older than the turtle crown clade, which arose around 160 million years ago, Proganochelys is anatomically primitive in many ways. But with a fully formed shell that includes a carapace dorsally and plastron ventrally, Proganochelys had already achieved an essentially modern turtle body plan. It thus cannot serve as the transitional form we need to more securely fasten the turtle crown clade to one of the other branches of the reptile tree. Science finally caught a break in 2008 with the discovery and description of Odontochelys semitestacea from the Late Triassic of China. Approximately ten million years older than Proganochelys, Odontochelys bears a fully formed plastron, but the carapace is reflected almost exclusively in its distinctively widened ribs. These ribs closely resemble those of baby modern turtles whose shells are just beginning to develop. Although Odontochelys does not provide many obvious clues as to where turtles belong within the larger radiation of reptiles, it certainly lends valuable insights as to what we might expect to uncover in the even deeper portions of the turtle stem lineage.
About the time that Odontochelys was published, I arrived as a post-doctoral researcher at Yale University where I began to work closely with Dr Tyler Lyson, then a Ph.D. student and now Assistant Curator of Vertebrate Paleontology at the Denver Museum of Science and Nature in Colorado. Tyler and I both were interested in the early history of reptiles and the origin of turtles, and having read the description of Odontochelys, we quickly recognised the potential importance of an even older fossil reptile named Eunotosaurus africanus. Eunotosaurus was first described in 1892 by H. G. Seeley of the Natural History Museum in London based on specimens collected from rocks of the Karoo Basin in South Africa that are currently dated to about 260 million years old — approximately 40 million years older than those producing Odontochelys. The turtle-like shape of its trunk and its distinctively broadened ribs, make Eunotosaurus highly congruent with what might be expected for the earliest chapters of turtle history based on the morphology expressed by Odontochelys. So with the generous support of our colleagues in South Africa, where almost all of the known specimens of Eunotosaurus reside in museum collections, we set to work. Especially Tyler, who took the lead on a series of studies that became the core of his award-winning dissertation. These studies aimed to determine whether the turtle-like appearance of the Eunotosaurus post-cranial skeleton is superficial only, and thus evolutionarily misleading, or does it reflect the same evolutionary transformations that constitute the structural groundplan of the modern turtle shell. The resultant data strongly support the latter conclusion with Eunotosaurus sharing a number of unique turtle features. These not only include the unique way that turtles widen their ribs with sub-dermal bone streaming out from the perichondral collar of the rib’s endochondral core to produce a T-shaped structure in cross section, but also the highly derived mechanism through which modern turtles ventilate their lungs. Eunotosaurus thus sheds light on the earliest history of turtle-specific transformations – transformations that have eluded biologists since Darwin established that such a history must exist. Discerning what Eunotosaurus means for the position of turtles within the greater radiation of reptiles, however, required a better understanding of its skull.
The vertebrate skull is a complex anatomical system packed full of clues for reconstructing evolutionary history. The skull of modern turtles, though not as strikingly bizarre as their post-cranial skeletons, still bears a number of distinguishing features. Turtles, for example, have a shortened preorbital face, they bear little ability to move the individual cranial bones relative to each other (cranial kinesis), and they famously lack teeth – both along the margins of their jaws and on the palatal roof of their oral cavity. Uncontroversial stem turtles such as Proganochelys and Odontochelys have done much to illuminate where many of these modern cranial features find their origin along the turtle stem lineage. We now have, for example, clear evidence that turtles lost their teeth in two stages, with the marginal teeth being lost somewhere in the tree space between Odontochelys and Proganochelys, followed by the palatal teeth in the tree space separating Proganochelys and the turtle crown clade.
One of the most distinctive features of the turtle skull among modern reptiles may or may not find its evolutionary origin along the turtle stem lineage. This feature is a complex of dermal bones set behind the eye that forms a completed roof and lateral wall of the temporal fossa – the chamber housing the adductor muscles responsible for closing the jaws. It was long thought that this “anapsid” condition reflected the primitive condition for reptiles and was thus strong evidence that the origin of turtles was to be found among other early anapsid reptiles, many of which are today classified as parareptiles. If, however, the molecular-based solution is correct and turtles are nested inside the saurian radiation, where the temporal fossa is pierced by an upper and lower opening (the upper and lower temporal fenestrae, respectively – an arrangement known as the “diapsid” condition), then the anapsid skull of turtles must be secondarily evolved.
Although this solution is by far the most popular among biologists and paleontologists alike, there has never been any direct paleontological evidence of the inferred transformation between an ancestral stem turtle with a diapsid skull and the anapsid morphology of modern turtles (both Odontochelys and Proganochelys express the anapsid structural plan).
It was within this context that I began to study the cranial anatomy of Eunotosaurus using high-resolution computed tomography (CT). The small amount of previous work on the known skulls of this animal emphasized two points: Their overall primitive nature and probable taxonomic affinity with parareptiles, and the absence of any cranial evidence supporting a unique relationship between Eunotosaurus and turtles. Modern imaging technology provided me access to cranial details that previous workers were denied, and as it turns out, this made all the difference. Realising that difference however, was no simple task, exacting its pound of flesh in the form of more than three years digitally individuating each bone in two of the best preserved skulls.
The product of that effort was a suit of cranial features supporting Eunotosaurus as the earliest stem turtle. Examplar turtle features include a shortened face, a unique bracing of the otic capsule against a bone supporting the middle ear called the quadrate, and a rare ossification of the cartilages deep to the eye to form a bony wall of the anterior braincase. The cranial signal is thus congruent with that of the postcranial skeleton in its support of Eunotosaurus as the earliest member of the turtle lineage. But what does the skull reveal about the position of turtles in the larger reptile tree of life?
Unlike Proganochelys and Odontochelys, Eunotosaurus is not strictly anapsid. Its adductor chamber is exposed through what may be justifiably interpreted as a diapsid-like lower temporal fenestra in the cheek. The chamber’s roof, however, is closed, lacking the upper temporal fenestra that, when present, serves as an unambiguous indicator of diapsid ancestry. Occupying the space where one would normally find the upper temporal fenestra in a saurian skull is an elongate bone known as the supratemporal. Purely out of curiosity, I rendered away the digital version of the supratemporal just so that I check the architectural details of how the dermal roof of the temporal fossa was constructed deep to that bone. In doing so, I was faced with a circular opening bounded by the same bones defining the diapsid upper temporal fenestra in a saurian skull. Could this opening represent a vestige of that diapsid window?
My colleagues and I constructed just that argument buoyed by our observations that no such opening is present deep to the supratemporal in either early stem-group mammals or parareptiles. The importance of this supplementary evidence quickly diminished when we were shown a newly prepared juvenile specimen of Eunotosaurus in the collections of the South African Museum in Cape Town. The specimen expresses enlarged versions of both the lower and upper temporal fenestra, and clearly establishes Eunotosaurus as a diapsid, saurian reptile in a privileged relationship with turtles. Placing the growth trajectories inferred between the juvenile and adult specimens of Eunotosaurus within a phylogenetic context provided the first empirically based model for how modern turtles acquired their anapsid skulls from a diapsid ancestor. Thus, a combination of fossils, molecules, technology, and a bit of both hard work and luck has removed turtles from the nebulous shadow that obscured their evolutionary origin for well over 150 years.
The author:
Dr Gaberiel Bever is Assistant Professor of Anatomy at the New York Institute of Technology.