Dark energy: the cosmic frontier
7 Jan 2016 by Evoluted New Media
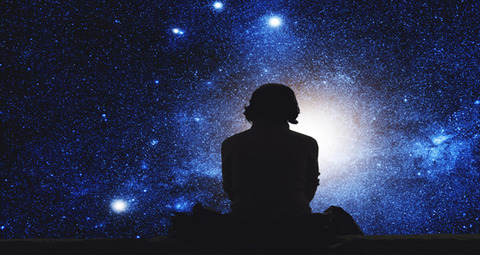
Seventeen years ago astronomers announced a remarkable observation – the Universe’s expansion is accelerating.
Seventeen years ago astronomers announced a remarkable observation – the Universe’s expansion is accelerating. If the Universe is made only of matter, and the only force acting on large scales is gravity, then the Universe’s expansion should be doing exactly the opposite. The discovery (awarded the Nobel Prize for Physics in 2011) shook our cosmological model to the ground; the job of the science community was to build it back up...
There are a number of proposed explanations for the phenomenon dubbed dark energy. The simplest from a theoretical point of view (but not conceptually) is the idea of a cosmological constant – a simple constant term in Einstein’s equations of General Relativity that would naturally explain the acceleration. In physical terms, this would equate to a bizarre property of empty space, a type of vacuum energy that would cause space itself to expand.
At the other extreme of possible explanations for Dark Energy are modified gravity models. Einstein’s theory of General Relativity has passed stringent tests at solar-system scales. But if this theory breaks down on very large scales or at very low densities, then perhaps this acceleration is an illusion that stems from an incomplete theory of gravity. But which is it? How can we tell? Different explanations of dark energy predict slightly different expansion histories of the Universe, so the first task is to measure how the expansion has changed with cosmic time.
For that we need a standard ruler in the universe, something whose size is fixed. Thankfully, Nature was kind enough to provide one. Shortly after the big bang the universe was so hot and dense that all matter existed in the form of plasma, and behaved like a fluid. This fluid wasn’t all of uniform density – some parts were denser than others, and these small gradients set up pressure waves in the fluid that propagated at the speed of sound; and continued to propagate while the universe was dense enough to support them. But the universe expanded, and the density decreased. At one point – about 350,000 years after the Big Bang – the density was too low for sound waves to propagate, and these ripples became frozen in the matter distribution of the universe. Crucially, using simple physics and observations of the cosmic microwave background, we can compute the size of this wave in the early Universe – and by measuring its size at later times, we can directly trace the expansion of the universe.
These ancient waves are called baryon acoustic oscillations (BAO). ‘Baryon’ because they exist due to the interaction of baryonic (normal, i.e., not dark) matter with radiation; ‘Acoustic’ because they are pressure driven, like sound; and ‘Oscillations’ because they are waves. The BAO size shows itself in the spacial distribution of galaxies. Galaxies tend to form where the matter density is largest, and we expect to see an excess of galaxies at the crests of density waves. In practical terms, there will be a preferred distance at which galaxies tend to be separated. The magic number is around 500 million light-years. Because of the regularity of those ancient waves, there is a slightly increased probability that any two galaxies today will be separated by about 500 million light-years, rather than 400 million or 600 million. By measuring the angle on the sky subtended by this standard-ruler in galaxies at different distances from us, we can compute the distance to those galaxies – and build up a cosmic distance ladder that traces the expansion history of the Universe.
The quest for measuring the BAO at large distances and with increasing precision is driving the most ambitious astronomical surveys in history. The latest, called Baryon Oscillations Spectroscopic Survey (BOSS) and part of the Sloan Digital Sky Survey III (SDSS-III), produced a monumental three-dimensional map of the Universe using the positions of nearly 1.5 million galaxies. BOSS was a five-year observational campaign at the Sloan Telescope, and it measured the accurate positions of a few thousand galaxies every night. Whereas making a two-dimensional map of the sky is easy, the third dimension – or distance from us – is harder to get.
In an expanding universe, there is an exact relationship between distance and the velocity of a galaxy with respect to us. Galaxy redshift surveys effectively measure the recession velocity of a galaxy by measuring its redshift – the shift in the spectrum of a galaxy towards the red due to its motion. Within a cosmological model, redshifts can then be trivially interpreted as physical distances. Whereas a few decades ago obtaining the spectrum of a galaxy was laborious, modern day and future instruments can be fed hundreds to thousands of individual optical fibres, allowing us to map the universe at astounding speed. Using galaxy redshifts to detect the baryonic acoustic oscillations, BOSS measured the expansion history of the universe with astounding clarity, revealing an expansion dominated by the cosmological constant, or a constant vacuum energy. However, there is a weakness to limiting the analysis of dark energy to the expansion history. If one is prepared to change the laws of gravity, one can always come up with a theory that matches the measured expansion without the need for dark energy. Under such propositions, the laws of gravity deviate from general relativity on very large scales.
Gravity causes structure to grow – small fluctuations in density in the early Universe gave rise to the largest structures in the universe today (vast over-densities of galaxies) simply due to gravity. Crucially, this growth allows us to test the laws of gravity. If gravity behaves as Einstein suggested it did, even at these colossal scales, we know how fast structure should be growing in the universe. Gravity and dark energy affect the motions of galaxies in different ways. By and large, the motion of a galaxy is set by the expansion of the universe (hence the relevance of the BAO measurements). But with a large and detailed enough map, we can also detect the velocity component due to growth: due to gravity.
To understand how, let us remember that galaxy redshift surveys produce a redshift map. On one direction and one direction alone – that is along our line of sight – it gives us a recession velocity, or redshift. As galaxies continue to follow the growth of large structures, their velocity due to this motion is added to their redshift and galaxies appear to be slightly closer or further away than they really are. This effect distorts the three-dimensional maps in a very peculiar way, squashing them along the line of sight. A faster growth of structure results in a more prominent ‘squashing’ signal, giving the name to this technique – redshift space distortions. By measuring these distortions, we can measure the rate at which structure grows and directly test gravity.
BOSS, with its large volume and number of galaxies, set a rigorous test of Einstein’s theory of gravity at these very large scales. And what is outstanding is that it still seems to work. This is a profound statement about the physical nature of our universe, and one that can’t be made lightly. When combined with the measured expansion history of the Universe using the baryonic acoustic oscillations, BOSS reveals a universe that is currently dominated by a cosmological constant and is governed by General Relativity. Whereas most quantum field theories in particle physics predict a non-zero value for vacuum energy, unfortunately they tend to be around 120 orders of magnitude larger than the measured value of the cosmological constant.
The nature of dark energy continues to be the greatest mystery in modern day cosmology – and potentially particle physics – and whatever the answer, it will prompt a revolution in our fundamental understanding of the governing laws of the Universe.
The author: Dr Rita Tojeiro is a Research Fellow at the School of Physics and Astronomy at the University of St Andrews. She is also a winner of the 2015 L’Oréal-UNESCO UK & Ireland Women In Science Fellowships.