The venom that packs a punch for cancer
7 Dec 2015 by Evoluted New Media
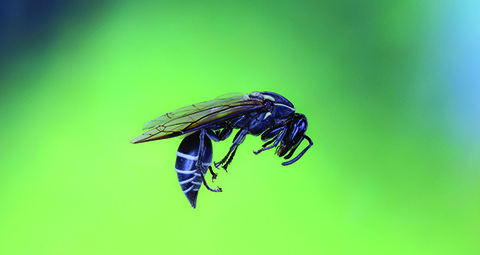
Animal venoms have exciting potential applications as new forms of chemotherapy that can attack cancerous cells. Dr Anders Aufderhorst-Roberts discusses his involvement in recent work aiming to understand the mechanism behind an anti-cancer molecule found in wasp venom
Animal venoms have exciting potential applications as new forms of chemotherapy that can attack cancerous cells. Dr Anders Aufderhorst-Roberts discusses his involvement in recent work aiming to understand the mechanism behind an anti-cancer molecule found in wasp venom
Each year more than 14 million people are diagnosed with cancer and the number of new cases is expected to rise by 70% over the next two decades. Many different treatments have been devised to kill cancerous cells. Some block DNA replication in the cell, some directly damage the DNA while others prevent cell division. However all of these therapies remain massively invasive: they require catheters to deliver the chemotherapies and often have to be combined with radiotherapy and surgery. Also, many current drugs are nonselective – they kill healthy cells as well as cancerous cells. Cancer treatment remains very unpleasant and the search for new and better chemotherapy drugs is a major goal for biotechnology researchers.
Many researchers have found that taking inspiration from nature can be a fruitful way of finding new treatments. Animal venoms in particular have been particularly useful in this regard and over the last ten years researchers have found chemical compounds that have anti-cancer potential in a diverse range of venomous creatures including various species of snake, frog, scorpion, toad, bee and wasp.
Hidden amongst the many toxic and often deadly substances that make up these venoms it seems, are a few isolated molecules that happen to be harmful to cancerous cells. But one fundamental problem remains. We need to be sure that the active chemical in the venom effectively kills cancerous cells but doesn’t harm healthy cells. Creative solutions have been proposed, for example some researchers have suggested attaching the active chemical to a nanoparticle that selectively delivers it to the cancerous tumour site. But this adds an additional layer of complexity to an already complex problem.
The discovery of polybia MP1 was a big step forward in this endeavour. MP1 is a peptide found in the venom of the Brazilian wasp Polybia paulista. The wasp is highly aggressive and causes several cases of severe envenomation a year. The MP1 peptide is just one of hundreds of molecules present in the wasp’s venom. Previous studies by other research groups showed that the MP1 peptide, (when used in isolation) appeared to destroy both bladder and prostate cancer cells while leaving healthy cells intact. A first step in developing the MP1 as a useful drug is to understand how it works.
This is where our research came in. We wanted to understand the mechanism of how the MP1 peptide led to cancer cell death and to do this we decided to focus our attention on the cell membrane. All cells need the membrane to obtain energy, get rid of waste and communicate and so it’s a region of the cell where a great deal of biological activity happens. As a result it’s also an important aspect of many diseases – currently over 60% approved targets for drugs involve the cell membrane. Significant damage to the membrane will cause cell death.
There’s also some very clear known differences between the membranes of healthy cells and the membranes of cancerous cells. A healthy cell has what we call an asymmetric membrane. The molecules facing the inside of the cell are different to those facing outwards. But when the cell becomes cancerous many of the molecules that face inwards move to the outside and are exposed to the outside environment. Two molecules in particular, PE and PS, are much more represented on the outside of cancerous cells. So perhaps these two molecules somehow allow or encourage the MP1 peptide to damage the cancerous membrane and cause the cell to die. It’s not enough to expose the venom molecules to cancerous cells – because it could be argued that any number of factors could lead to the cell death, cells are complicated systems after all. We needed to simplify the problem. To do this, with our collaborators in Brazil, we prepared a range of different ‘minimalised' cells, basically just an empty shell consisting of a simple lipid membrane and nothing else. We prepared some of these minimal cells with both of the candidate molecules, PS and PE, others with just one of the two molecules and finally another set where neither molecule was present. The minimal cells were placed in an environment of different sized fluorescent dyes so that if the membrane was at all damaged the dyes would visibly leak inside the minimal cell.
Hidden amongst the many toxic substances that make up venoms, a few isolated molecules are harmful to cancerous cells
The results were very interesting. Firstly, we found that when neither of the two molecules, PS and PE, were present the membrane stayed largely intact because none of the dye leaked into our minimal cells. When either or both of the two molecules were present leakage could clearly be seen with the most leakage being observed when both were present. Interestingly, through using spectroscopy, we also observed that the MP1 peptide appeared to bind to the surface of the membrane when one of the two molecules PS was present. From this we could theorise that the PS molecules help the venom to bind to the membrane whereas the PE molecules help the venom punch pores in the membrane, causing the dye to leak inside. But we wanted further validation of this theory – with the technique described above it wasn’t possible to actually see the pores in the membrane, but only to infer that they existed by the fact that leakage occurred. We also couldn’t measure the size of the holes being formed, although using different types of dye did give us a rough indication of when smaller or larger holes were forming.
In Leeds we used atomic force microscopy to visualise the membrane surface. Atomic force microscopy is a technique that essentially ‘feels’ rather than ‘sees’ the surface. To do this it involves an ultra-sharp needle (the radius on the end is just tens of atoms across) attached to a flexible cantilever. The needle is run across the surface of the membrane and any bumps or holes in the membrane cause the needle to move up or down. From this a three-dimensional picture of the surface can be made. Again, we prepared membranes with either both PE and PS, just one of them or none of them and then added the MP1 peptide. Our results were strikingly similar: when both PE and PS were present, large pores clearly appeared on the surface after just a few minutes, the pores growing gradually over time. Much smaller pores also appeared when just one of the two molecules were present in the membrane. When only PS was present, tiny bumps were also observed on the surface suggesting, as before, that the PS molecule helps the MP1 peptide to stick to the surface of the membrane.
So there are a few take-home messages from our results. Firstly, we can say that the venom molecule MP1 appears to attack the membrane and that it needs two key molecules PE and PS to work effectively. PS helps the venom to stick to the surface while PE causes it to punch through the membrane and form a pore that ultimately leads to the death of the cancerous cell. This is an exciting result because we have shown for the first time the mechanism for how cancerous cells might be selectively killed by directly attacking their membrane. This mechanism could be used as a potentially new form of chemotherapy or at least guide the development of other treatments.
But more work is needed. We don’t, for example, know that MP1 only targets the membrane and its potency could be related to other factors too. We also need to see how MP1 could be made more effective in clinical studies, for example by modifying its chemical structure to make it even more selective and even more potent. There could also be the potential to use it in combinations with other therapies that attack the inside of the cell making a for a potentially more powerful combination therapy.
The author:
Anders Aufderhorst-Roberts obtained his PhD in Biological and Soft Matter Physics from the University of Cambridge in 2013. He is currently a Postdoctoral Research Fellow at the University of Leeds, where he uses a range of advanced atomic force microscopies to materials including soft polymers and biomembranes.