Characterising the future of medicine
13 Oct 2015 by Evoluted New Media
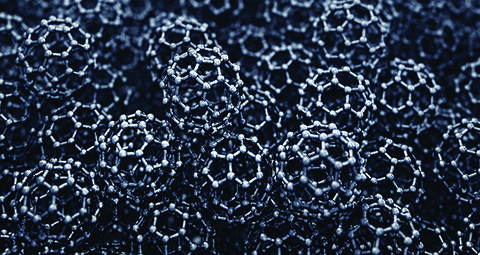
Nanotechnology offers great promise for the improvement of the treatment of a number of diseases. However, access to the expertise and methodologies required to characterise new nanomaterials is limited. Now, the new European Nanotechnology Characterisation Laboratory is set to change this as it offers translational resources to developers across Europe.
Nanotechnology offers great promise for the improvement of the treatment of a number of diseases. However, access to the expertise and methodologies required to characterise new nanomaterials is limited. Now, the new European Nanotechnology Characterisation Laboratory is set to change this as it offers translational resources to developers across Europe.
Nanomedicine, the application of nanotechnology within medicine, is already contributing to health on a global scale with a number of nanoparticle-based medicines already employed in the clinic. In 2013 there were 45 nanoparticulate therapeutics approved for use with many more currently in phase II and phase III clinical trials1. However, within many academic and industrial research activities, the potential benefits can be overstated and proposed technologies can be inferred to be very close to application when clear hurdles still exist in their translation to market.
There are a wide range of definitions relating to nanotechnology and there is yet to be a global consensus on how it should be defined. The National Nanotechnology Initiative (NNI), a US federal government programme has stated: “Nanotechnology is the understanding and control of matter at dimensions between approximately 1 and 100nm, where unique phenomena enable novel applications...Encompassing nanoscale science, engineering, and technology, nanotechnology involves imaging, measuring, modelling, and manipulating matter at this length scale.”2 Other investigators and authorities set the size cut-off for definition of a nanomedicine at below 1000nm and ultimately it is the therapeutic, toxicological or posological advantages that are the most important drivers.
The upper size limit has its roots within the inorganic study of small particles as it essentially outlines the size range at which there is often transition between bulk and non-bulk properties3 of materials (usually metals). The limitation of sizes to the 1-100nm range, however, is not meaningful as new and highly valuable interactions of materials with complex biological systems have been observed at sizes considerably above the 100nm upper limit. A broader and more inclusive definition is used by the European Technology Platform on Nanomedicine (ETPN): “Nanomedicine is the application of nanotechnology to health. It exploits the improved and often novel physical, chemical, and biological properties of materials at the nanometric scale”4. Within industry, a general agreement has been voiced at many conferences that the size range is not the focus but rather the improvement and optimisation of material properties drives patient benefits. As such, a broader range of dimensions, from 1-1000nm is typically considered the nanomedicine range. For context, the width of human hair is approximately 50,000 to 80,000nm.
Many different types of nanoparticles exist including, but not limited to; inorganic nanoparticles (e.g. gold, silver and silica), polymer based nanoparticles (e.g. dendrimers, polymer-drug conjugates) and lipid-based nanoparticles. The variety of classes of nanomaterials, in addition to the variation of nanoparticle physical and compositional characteristics, is perhaps the greatest advantage of nanomedicines but may also serve as one of the greater challenges in their rational design. These characteristics greatly influence their utility for drug delivery as they influence how they interact with biological systems and a greater understanding of these relationships is urgently required for nanomedicines to reach their full utility. A large percentage of drugs are not well absorbed due to poor solubility in intestinal fluid as well as poor permeation through the intestinal epithelium. Nanoformulation can be used to improve the dispersion of poorly water soluble drugs as well as enhance their transport across the intestinal barrier. In addition to application for improved oral delivery, nanomedicines have also demonstrated improved safety over conventional medicines by targeting their therapeutic payload specifically to diseased areas of the body via passive or active mechanisms.
The behaviour of nanomaterials has been demonstrated to differ considerably from larger bulk material not just in their absorption and targeting, but also their interactions with cells in general5. Importantly, the single biggest driver for application of nanotechnology in drug delivery is the improvement of the safety of medicines and this has been explicitly demonstrated for a number of commercialised nanocarrier medicines. However, as for all medicines, nanomedicines require a robust assessment of safety prior to human applications and there remain uncertainties surrounding the potential risks posed to human health and environment. The size of nanomaterials influences their toxicity5 and this has been consistently demonstrated for nanomaterials encountered in workplace environments (e.g. carbon black, polystyrene, titanium dioxide and silver)6-9.
Nanomaterials vary considerably with respect to their composition, charge, surface area, solubility, crystal structure, surface chemistry and shape. Many nanomaterials have been shown to interact with the immune system by either stimulating or suppressing various responses10,11 through their interaction with proteins found within blood12-15. Many nanomaterials interact with serum proteins such as opsonins (e.g. antibodies, complement) and are rapidly taken up into cells of the mononuclear phagocyte system (e.g. macrophages)14. Interactions with serum proteins renders some nanomaterials antigenic and it has been suggested that functionalisation (e.g. with growth factors, receptors) may induce neutralising antibodies that also recognise the body’s own molecules with implications similar to those for biotechnology-derived therapeutics16,17.
Immune cells recognise nanoparticles based on their surface properties and core composition and mount inflammatory responses but many of the molecular events are poorly understood18. In general, positively charged nanomaterials are more likely to induce inflammatory reactions than negatively charged or neutral materials. For example, positively charged 4.5 polyaminoamine (PAMAM) dendrimers do not cause human leukocytes to secrete cytokines19 but negatively charged liposomes induce secretion of cytokines such as IL-2 and IFN?20. Clearly, there are toxicological consequences for small molecule drugs as well, and drug research in recent years has made great progress in understanding the critical molecular triggers so that toxicity can be mitigated early in development. There is now a need for similar understanding in relation to nanomedicines and the EU-NCL will be at the forefront of research in this area to help facilitate translation of safe and effective nanomedicines.
Given the increasing development of nanomaterials across Europe a need has been identified to begin to regulate the preclinical evaluation of novel engineered nanomaterials as well as provide a platform for the translation of these materials into clinical studies. The European Commission's Scientific Committee on Emerging and Newly Identified Health Risks acknowledge that nano-specific test systems and assessment schemes require development21 and in February 2013 specific considerations for biodistribution and biocompatibility (e.g. uptake by reticuloendothelial system) of liposomes, block co-polymer micelles and iron nanoparticles were published22. The regulatory challenges for the development of nanomedicines in Europe are complex and have been summarised elsewhere23. Complete translation from initial nanomaterial design to the market is long and requires a number of approval steps that involve increasing data requirements. More than €600 million were committed in the European Commission’s FP7 programme to develop concepts and nanomaterials for the health care sector. Moreover, approximately 6500 patents filed across Europe are in need for further translation into products and more than 500 European small to medium sized enterprises (SMEs) are actively engaged in development nano-based health care products. Importantly, SMEs lack the resources of larger pharmaceutical companies and this can preclude effective translation of products. Therefore, EU-NCL will interface with regulatory agencies and product developers to facilitate this translation.
Single biggest driver for application of nanotechnology in drug delivery is the improvement of the safety of medicines
The currently ongoing European framework programme “Horizon 2020” has a strong emphasis on the translation of nanomaterials through to clinical trials and eventually the market by covering further development of the preclinical environment. In order to address these aspects, a number of projects have been funded to serve as translational hubs as suggested by the European Platform for Nanomedicine. These are; “ENATRANS” which will focus on measures needed to support the networking of stakeholders in nanomedicine, “Nanofacturing” and “Nanopilot” which are dedicated to the scale-up of the manufacturing of nanomedicines for clinical use. The EU-NCL is highly complementary to these other initiatives and will support the assessment of the quality and safety of nanomedicines during the preclinical phase.
EU-NCL shares the same ethos as the US Nanotechnology Characterisation Laboratory (US-NCL), which is funded by the US National Institutes for Health National Cancer Institute. US-NCL was created to aid in the translation of novel nanomedicines for the treatment of cancer and during its tenure has performed characterisation on over 400 nanomaterials serving as a national resource and knowledge base for cancer researchers by facilitating nanomaterial development. Importantly, the US-NCL is a partner in the creation of the EU-NCL along with seven other core members including; Commissariat à l’énergie atomique et aux énergies alternatives (CEA, France), European Commission's Joint Research Centre (JRC, Italy), Trinity College Dublin (Republic of Ireland), Stiftelsen SINTEF (Norway), University of Liverpool (UK), Eidgenoessische Materialpruefungs- und Forschungsanstalt (EMPA, Switzerland), Gesellschaft für Bioanalytik Münster (Germany).
The lack of an organisation at the EU-level that would provide adequate regulatory preclinical characterisation of nanomaterials slows down the translation of promising nanomaterials for medical use into clinical products. Currently, researchers have to gather preclinical data from a multitude of non-integrated providers. The major ambition of the EU-NCL is therefore to tackle that obstacle by providing an open-access EU-wide characterisation infrastructure and maintain Europe as internationally competitive in nanomedicine characterisation. EU-NCL will offer a unique integrated solution ensuring access to high quality data, experience, and facilities throughout Europe for a large range of medical applications.
The organisation of EU-NCL is different to that of the US-NCL. EU-NCL is a multi-centre infrastructure which aims to support its ambition to overcome current fragmentation and to improve quality and efficiency of translation. The involvement of multiple analytical centres will guarantee direct access to different domains in the nanomedicine communities and other stakeholders, while maintaining the bandwidth to engage with Europe’s most promising nanomedicines. Submissions from nanomedicine developers will be reviewed by a core expert team (CET) which will make decisions on which materials enter the analytical cascade based on information from the submitting centre such as reproducibility of the production of the nanomaterial, envisaged route of administration and a demonstration of efficacy against the relevant disease or target.
EU-NCL will develop and standardise analytical cascades and procedures for the characterisation of engineered nanomaterials that will be made available to the larger nanomedicine community in order to ensure parity between various characterisation efforts. The utility of a multi-centre infrastructure ensures that the analytical process is constantly assessed and refined as necessary since each partner has specific expertise in complementary methods and protocols as well as receiving fresh input from other initiatives. This goal is supported by the inclusion of a “technology scouting” workpackage aimed at identifying new techniques or biological markers that may enhance the preclinical characterisation of nanomaterials. These new methodologies will be rigorously assessed for their applicability as well as their cost effectiveness prior to incorporation into the analytical cascade.
Continuous enhancement and refinement of the analytical cascade will also include liaising with various regulatory agencies (e.g. EMA, National Competent Authorities) in order to ensure EU-NCL stays “regulatory-relevant” as well as advising national agencies and the European Medicines Agency (EMA) on developments within the scientific community. EU-NCL has the ambition and expertise to liaise with the European regulatory bodies and to act as the European reference infrastructure in nanoparticle characterisation.
This will alleviate many of the current regulatory challenges regarding clinical evaluation and market authorisation of nanomedicine products. The proposed integration of national infrastructures into a unique European infrastructure is recognised by the European and American nanomedicine stakeholders as a first but major step towards the harmonisation among EU Member States but also between the EU and the USA.
In summary, the development of the EU-NCL will serve to support nanomedicine development throughout Europe and translation of these new products through to clinical utility. A call for submission of the first materials launched in summer 2016.
References:
- Schütz CA, Juillerat-Jeanneret L, Mueller H, Lynch I, Riediker M. Therapeutic nanoparticles in clinics and under clinical evaluation. Nanomedicine. 2013;8(3):449-67.
- http://www.nano.gov/nanotech-101/nanotechnology-facts [29/07/15].
- Ramsden JJ, Julie F. The Nanoscale. Nanotechnology Perceptions. 2009;5:3-25.
- ftp://ftp.cordis.europa.eu/pub/nanotechnology/docs/nanomedicine_visionpaper.pdf [29/07/15].
- Johnston H, Brown D, Kermanizadeh A, Gubbins E, Stone V. Investigating the relationship between nanomaterial hazard and physicochemical properties: Informing the exploitation of nanomaterials within therapeutic and diagnostic applications. Journal of controlled release : official journal of the Controlled Release Society. 2012;164(3):307-13.
- Ferin J, Oberdorster G, Penney DP. Pulmonary retention of ultrafine and fine particles in rats. American journal of respiratory cell and molecular biology. 1992;6(5):535-42.
- Li XY, Brown D, Smith S, MacNee W, Donaldson K. Short-term inflammatory responses following intratracheal instillation of fine and ultrafine carbon black in rats. Inhalation toxicology. 1999;11(8):709-31.
- Brown DM, Wilson MR, MacNee W, Stone V, Donaldson K. Size-dependent proinflammatory effects of ultrafine polystyrene particles: a role for surface area and oxidative stress in the enhanced activity of ultrafines. Toxicology and applied pharmacology. 2001;175(3):191-9.
- Gaiser BK, Fernandes TF, Jepson M, Lead JR, Tyler CR, Stone V. Assessing exposure, uptake and toxicity of silver and cerium dioxide nanoparticles from contaminated environments. Environmental health : a global access science source. 2009;8 Suppl 1:S2.
- Dobrovolskaia MA, Aggarwal P, Hall JB, McNeil SE. Preclinical studies to understand nanoparticle interaction with the immune system and its potential effects on nanoparticle biodistribution. Molecular pharmaceutics. 2008;5(4):487-95.
- Dobrovolskaia MA, McNeil SE. Immunological properties of engineered nanomaterials. Nat Nanotechnol. 2007;2(8):469-78.
- Goppert TM, Muller RH. Polysorbate-stabilized solid lipid nanoparticles as colloidal carriers for intravenous targeting of drugs to the brain: comparison of plasma protein adsorption patterns. Journal of drug targeting. 2005;13(3):179-87.
- Leu D, Manthey B, Kreuter J, Speiser P, DeLuca PP. Distribution and elimination of coated polymethyl [2-14C]methacrylate nanoparticles after intravenous injection in rats. Journal of pharmaceutical sciences. 1984;73(10):1433-7.
- Gref R, Minamitake Y, Peracchia MT, Trubetskoy V, Torchilin V, Langer R. Biodegradable long-circulating polymeric nanospheres. Science. 1994;263(5153):1600-3.
- Deng ZJ, Liang M, Monteiro M, Toth I, Minchin RF. Nanoparticle-induced unfolding of fibrinogen promotes Mac-1 receptor activation and inflammation. Nat Nanotechnol. 2011;6(1):39-44.
- Kivisakk P, Alm GV, Fredrikson S, Link H. Neutralizing and binding anti-interferon-beta (IFN-beta) antibodies. A comparison between IFN-beta-1a and IFN-beta-1b treatment in multiple sclerosis. European journal of neurology : the official journal of the European Federation of Neurological Societies. 2000;7(1):27-34.
- Swanson SJ, Ferbas J, Mayeux P, Casadevall N. Evaluation of methods to detect and characterize antibodies against recombinant human erythropoietin. Nephron Clinical practice. 2004;96(3):c88-95.
- Aggarwal P, Hall JB, McLeland CB, Dobrovolskaia MA, McNeil SE. Nanoparticle interaction with plasma proteins as it relates to particle biodistribution, biocompatibility and therapeutic efficacy. Advanced drug delivery reviews. 2009;61(6):428-37.
- http://ncl.cancer.gov/120406.pdf.
- Tan Y, Li S, Pitt BR, Huang L. The inhibitory role of CpG immunostimulatory motifs in cationic lipid vector-mediated transgene expression in vivo. Human gene therapy. 1999;10(13):2153-61.
- http://ec.europa.eu/health.
- http://www.ema.europa.eu/ema/index.jsp?curl=pages/regulation/general/general_content_000564.jsp&mid=WC0b01ac05806403e0.
- Bremer-Hoffmann S, Amenta V, Rossi F. Nanomedicines in the European translational process. European Journal of Nanomedicine2015. p. 191.
The author:
Neill Liptrott and Andrew Owen on behalf of the EU-NCL consortium.