Tapping microbial diversity with metagenomics has opened the door to more efficient biocatalysis approaches. The PolyModE project was an ambitious attempt to harness this technique for areas as diverse as medicine to food additives
Since 6000BC when the Sumerians first discovered the wonders of yeast in the production of beer, humanity has relied heavily upon on the use of microbes and their working tools – enzymes – from food production to cutting-edge medical applications. With the advent of molecular biology techniques shedding light on the role of enzymes, over the last century we have witnessed the increasing popularity of enzyme-produced food additives to improve the flavour or quality of a product, as well as innovative medical applications. Such biocatalysis presents a fast, safe and natural alternative to traditional chemistry, with the inherent specificity of an enzyme reaction producing less waste – a key benefit for industrial scale activities. Where productivity and efficiency are closely linked to profit, modern day commercial biotechnology strives to optimise biocatalysis, and this is achieved through two main routes:
- Sourcing novel enzymes – an incredible diversity of enzymes occur throughout living organisms, especially in microbes.
- Optimising the bioprocess – tweaking reaction conditions can vastly improve performance.
Armed with a diverse source of naturally occurring enzymes and the versatility to optimise reaction conditions, biocatalysis presents a powerful technique, especially for those reactions that are difficult to achieve through traditional chemistry.
Microbial diversity presents an abundant source of enzymes. However, much of this potential – estimated at over 99% – has remained unexplored, since many microbial species often fail to thrive under traditional culture conditions.
The advent of metagenomics in 1998
1 has revolutionised our ability to mine microbial diversity for novel enzymes (Figure 1). Bypassing the need for laboratory cultivation, this technique is instead based the extraction of genomic DNA directly from microbial communities residing in environmental samples such as soil, water or even the gut. This is genomics on a huge scale, and relies on the utility of next generation sequencing.
[caption id="attachment_37724" align="alignright" width="200"]
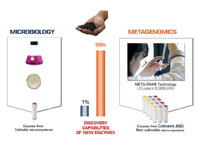
Figure 1: Sourcing novel enzymes through metagenomics. Directly isolating genomic DNA from microbes present in environmental samples with metagenomics, we can now tap 99% of microbial diversity for novel enzyme discovery. (Image courtesy of libragen)[/caption]
Once sequenced, large DNA fragments are then cloned into culturable host bacteria, to create metagenomic libraries ready for screening. These can be based on molecular approaches, searching for catalytic motifs within the genomic sequence. Alternatively, a high-throughput functional enzyme screen of recombinant proteins searches for catalytic activity and the subsequent database provides a useful resource for a range of applications.
When removed from their cellular environment, naturally occurring enzymes are not as efficient as they could be, presenting a major bottleneck in biocatalytic applications. Following enzyme discovery, multiple further stages are involved in subsequent bioprocess optimisation (Figure 2). Reaction conditions such as pH must be modified to mimic the
in vivo setting as closely as possible, thereby optimising enzyme performance. However, in some cases the reaction may also be limited by the enzyme itself, and genetic engineering technologies now make it possible to optimise the enzyme structure – a process known as directed evolution. Optimising conditions for enzyme performance within the industrial setting means that these enzymes can be put to use for real-world industrial applications.
Working towards the industrial scale production of clinical therapeutics and food additives, metagenomics and biocatalysis have recently been employed as part of the PolyModE (Polysaccharide Modifying Enzymes) project (www.polymode.eu). This European collaborative research effort, which ran from 2009 until April 2013, focused on developing polysaccharide modifying enzymes to optimise the use of six polysaccharides (also termed hydrocolloids): alginates, carrageenans, chitosans, glycosaminoglycans (GAGs), pectins, and xanthan gums. The PolyModE project was a success in identifying, characterising and optimising novel enzymes for the modification of all six hydrocolloids. As discussed in the following two examples, these discoveries have facilitated research into robust strategies for enzymatically-modified hydrocolloid production, ultimately at the industrial scale.
GAGs have many applications throughout medicine. Owing to a complex pattern of sulphation, their structures are highly functionalised and therefore connected to many biological properties, affecting conformation and ability to interact with biological factors (e.g. enzymes and growth factors).
By far the most intensely investigated GAG, heparin is commonly used as an anticoagulant – protecting against blood clots in hospital patients and blood transfusions. Sourced from animal tissues, natural GAGs pose all kinds of safety risks, especially in light of pathogen outbreaks such as bovine spongiform encephalopathy (BSE). Moreover, naturally occurring GAGs tend to exhibit extensive batch-to-batch variations, and uncertainty in a molecule’s activity can be particularly problematic in the clinical setting.
The complete synthesis of biologically active GAGs can overcome these limitations, but even this approach comes with its own set of challenges, and generating the full array of tailored sulphated variants remains a daunting task. Chemical sulphation techniques are difficult, time consuming and expensive, demanding many complex steps due to the lack of substrate selectivity. So why not instead make use of naturally occurring tools – enzymes that are both selective and efficient? Presenting an alternative to traditional chemistry, a major contributor to the PolyModE programme, CRO libragen employed its expertise in both metagenomics and biocatalysis for this very purpose.
[caption id="attachment_37725" align="alignleft" width="200"]
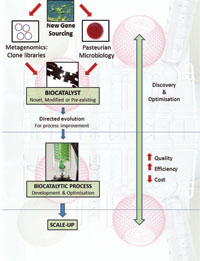
Figure 2: The perfect process. Creating an optimised biocatalytic process begins with the enzyme, be it novel, modified or pre-existing. Following process optimisation to ensure maximum efficiency and quality, the entire process is then scaled up for industrial application. (Image courtesy of libragen)[/caption]
Unknown to most, within the human digestive tract resides a community of bacteria well known for their ability to degrade GAGs, and recent genomic analysis has discovered that they carry an exceptionally high number of sulphatase encoding genes. Working on a heavily sulphated substrate GAG, these sulphatase enzymes remove selected sulphate groups, leaving a specific pattern behind and thereby generating a GAG specific to the enzyme involved. Through the discovery and optimisation of novel sulphatase enzymes derived from this resource, libragen’s objective was to facilitate the enzymatic synthesis of sulphated GAGs such as heparin.
In order to achieve this, metagenomic screening libraries were first constructed from this wealth of sulphatase genes derived from the human gut. The metagenomic library was screened firstly for basic sulphatase activity, and secondly for activity on a specific target substrate. As a result of this innovative approach, 10 novel sulphatases were identified from more than 20,000 screened metagenomic clones, and further research will shed light on their use in sulphated GAG production.
In addition to medical applications, hydrocolloids are also important additives in food, with the gelling agent carrageenan giving texture to cheeses and ice cream. But this substance is traditionally obtained from plants or algae – not the ideal solution when you consider that, for example, three quarters of the annually produced ca. 50,000 tons of carrageenan is extracted from only two species of red algae, which are becoming rare due to over-exploitation and global climate change.
As part of the PolyModE project, enzymes for producing superior quality carrageenan were isolated from the rare red algae, and optimised through directed evolution. These optimised enzymes could then be used to convert the inferior quality carrageenan from other, widespread red algal species into a superior quality product, ensuring a sustainable supply of this hydrocolloid for industrial application.
Biocatalysis yields many advantages for chemical synthesis, not least within pharmaceutical, cosmetic and food additive production. With ideals shifting towards reducing environmental impact, the rising emphasis on ‘green chemistry’ puts biocatalysis in the spotlight. Enzymes are highly efficient with excellent selectivity, increasing yields and producing less waste. Furthermore, by conducting reactions in water under ambient reaction conditions, both the use of organic solvents and energy input are minimised.
As demonstrated by the PolyModE project, research in this area is flourishing. Driven by powerful technologies such as metagenomics, these collaborations between academia and industry underline the future of ‘green chemistry’.
References
- Handelsman J., et al. Molecular biological access to the chemistry of unknown soil microbes: A new frontier for natural products. Chemistry & Biology 5 (10): R245–R249. 1998.
Authors
Daniel Auriol, MSc, PhD
, Chief Scientific Officer, and Cyrille Jarrin, Senior Scientist at libragen.
Contact
www.libragen.com.