Science on the cold front
6 Mar 2014 by Evoluted New Media
There are exciting areas of research that require extremely low temperatures, but getting down to near-absolute zero has its problems not least of which is the increasing scarcity of helium. Jeremy Good describes how new technology is getting around these issues Cryogenics is the study of low temperatures. Physicists and engineers are generating temperatures close to absolute zero to study the curious properties of atoms and materials that are apparent under these conditions. This work has led to the development of readily available and very powerful superconducting magnets which have opened up new industrial processes as well as areas of research, such as Nuclear Magnetic Resonance (NMR) and magnetic resonance imaging (MRI) techniques used by the medical profession and analytical laboratories. The original technique for getting down to such cold temperatures, both to generate high magnetic fields and to study materials, relied on liquid helium which boils at 4.2 Kelvin (-269?C) – just 4° above absolute zero. But helium is becoming scarcer and more expensive to obtain and liquefy, making this type of research increasingly expensive. In recent years, major research laboratories have had to temporarily shut down multimillion-pound facilities because of these shortages and the problem is set to get worse. Providing an alternative which doesn’t rely on a regular supply of helium is essential to addressing the problem. It will save time and money in the short term, and in the long term it may be the only option. The use of low temperatures can be divided into two main areas. Firstly, materials scientists and physicists conduct research at low temperature to understand the behaviour of materials, including superconductivity and superfluidity of helium itself. Nowadays, temperatures of less than 10 milliK above absolute zero are available in many laboratories, where the properties of graphene, carbon nanotubes, quantum dots, semiconducting and other nanoscale structures provide researchers with new physics to study. Quantum computing is an active and exciting field which requires these very low temperatures. The other principle use is in the generation of intense magnetic fields – which has become essential for NMR and MRI. Before superconducting magnets were developed in the 1960s, the maximum laboratory magnetic field was 2 Tesla and the field was generated with an iron core electromagnet. Only one or two laboratories worldwide could offer higher fields using watercooled coils of copper wire. To generate 14 Tesla in a 25mm bore required 2 megawatts of DC power, alternatively 4 Tesla could be generated in a 100mm bore with the same power source.
Major research laboratories have had to temporarily shut down multimillion-pound facilities because of shortages and the problem is set to get worseOnce superconducting magnets became available, higher fields could be generated at relatively low cost. Nowadays, most universities have access to high fields. Over the years, the maximum field from superconducting magnets has risen to over 20 Tesla. The size of magnets also increased as well, culminating in the 27 kilometres of magnets at CERN that form the Large Hadron Collider. Superconducting magnets offer two additional virtues. First, it is possible to have an exceptionally stable field. Secondly, the field can be made very uniform over a significant volume. This has led to the rapid development of NMR and imaging technologies which have been the most important advance in diagnostic medicine since the discovery and application of x-rays at the start of the 20th century. The magnetic fields are used to magnetise the atomic nuclei in samples and the magnetisation generates signals. These are manipulated to form images or yield information on chemical structure, revealing details of the properties of the sample being studied. Generating these strong magnetic fields requires superconducting magnets, which consist of materials that become superconducting at 9-18K and are normally operated at just 4K or 2K. Some years ago a new class of superconductors were discovered. These became superconducting at 100K or more. This raised the possibility of making magnets and other devices that could run with liquid air or nitrogen. However these High Temperature Superconductors (HTS) turned out to be difficult and expensive to produce and problematic to use. A metre of HTS conductor costs from $25 to $50 while an NbTi alloy conductor would cost just 20-30 cents. It is more cost effective to pay for the extra cost of refrigeration to achieve 4K and use NbTi rather than to pay the high cost of conductor and run at 77K – the boiling point of liquid nitrogen. This is hardly surprising as a typical laboratory magnet is likely to require 10 to 100 kilometres of conductor. There is a further intriguing factor. The properties of the HTS conductor improve as the temperature drops, with higher currents and higher maximum magnetic fields. It may well be that one of the first applications is to generate very high fields, in excess of 20 Tesla, with the HTS conductor running at 4.2K. So once again, liquid helium is required. Reaching and maintaining low temperatures has always involved helium, which must first be liquefied and transported. For magnets that run continuously, such as those in an MRI scanner which contains up to 2000 litres of liquid helium, a re-condenser or a helium recycling system is built in.
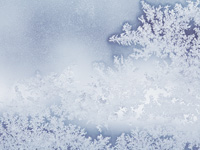