Milking it – Getting the most out of cheese aroma analysis
19 Jul 2012 by Evoluted New Media
Detecting aroma compounds in cheese challenges analytical equipment to the limit. Dr David Barden examines the latest advances that promise to uncover more about the variety of compounds released from this most popular and varied of foodstuffs
Cheese must be one of the most challenging things that come across the analyst’s bench. As a fermented milk product, it contains not just fats, proteins and salts, but also a range of small molecules (often referred to as volatile organic compounds or VOCs), the combination of which gives rise to the distinctive flavour of the cheese. These compounds are primarily the result of microbial and enzyme reaction during cheese ripening – explaining why unripened products such as cottage cheese don’t have any of the flavour complexity that you’d expect from a mature farmhouse Cheddar.
It’s easy to think that the flavour sensation that you experience when you eat foods such as cheese is just down to your tongue, but apart from the five basic taste sensations of sweet, salt, bitter, sour and umami (‘savoury’), your tongue’s got little to do with it. In actual fact, the aroma experienced when eating cheese depends largely on the VOCs released during chewing, which are drawn through the passages at the back of the throat (and then into your nasal cavity) when you exhale.
Over 600 of these volatile chemicals have been detected from cheese1, with 200 from Cheddar alone2 – indeed, in a study of 70 foods, Cheddar was found to have the greatest proportion of VOCs3. Because each chemical binds to the receptors in the nose in a different way, the description of cheese aromas needs a fairly extensive ‘lexicon’. Twenty-five descriptors are needed to cover common aroma components4, with examples including ‘buttery’, ‘fruity’ and ‘brothy’. However, another 19 are needed for more unusual flavour aspects – in Cheddar, for example, medium-chain fatty acids produce a ‘waxy/crayon’ component!5
Why does anyone bother to spend the time studying the chemical components of cheese aromas? The answer lies in the importance of cheese as a foodstuff – it’s long been valued as a means of preserving the nutrients of milk, and is now a global industry worth many billions of dollars annually. Cheese producers are naturally keen to ensure that their products are of a consistently high quality, so the chemical makeup of cheese is of prime importance. In addition, producers of cheeses with some sort of status protection (such as the ‘Protected Designation of Origin’ scheme operating in the EU) are also interested in knowing what makes their cheeses special – be that physical properties such as texture, or the chemical constituents that combine to produce their often distinctive aromas.
A good Brie should always have a detectable ammonia note, but keep it for too long, and it will let you know!Knowing what these chemicals are helps to shed light on the biochemical processes that give rise to them, which in turn can help to optimise the ripening process. This is particularly the case for some chemicals that are typical of the cheese variety, but which aren’t desirable elsewhere. As an example, p-cresol gives a characteristic ‘cowy/phenolic’ note to British farmhouse Cheddar, but the same aroma component has also been described as a defective attribute of cheeses in general6. Likewise, over-abundance of a particular component can make cheese smell bad – a good Brie should always have a detectable ammonia note, but keep it for too long, and it will let you know!
While the basic components of cheese (fat, protein, salts, etc.) are undoubtedly important, determining these is relatively easy by using standard food-analysis techniques such as gravimetric analysis, titration, potentiometry and Fourier-transform infra-red2. What is much trickier is detecting the VOCs present in the aroma profile, and then determining which of them is actually responsible for the perceived aroma (the general consensus is that only a small fraction contributes significantly1).
First of all, the analyst has the problem of separation – both of the VOCs from the cheese solids, and of the VOCs from each other. There are a number of methods2,7, which can be split into four broad groups:
- Direct extraction with an organic solvent or supercritical CO2, followed by evaporation
- Steam distillation, followed by extraction and concentration of the aqueous distillate
- Solid-phase micro-extraction, which adsorbs analytes from headspace or liquid onto a narrow fibre
- Headspace techniques, where the volatiles released by the food are captured, either in one go (static headspace) or continuously (dynamic headspace). The greater volume collected by dynamic techniques means that the gas has to be concentrated before analysis, but it has the advantage of allowing much greater sensitivity and allowing a wider range of analytes to be sampled.
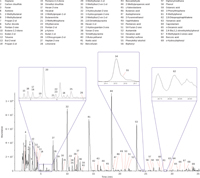
Extraction and distillation techniques are long-established, but they can also be rather labour-intensive and messy, and may lose the more volatile analytes during the evaporation step. Solid-phase micro-extraction is a relatively recent player, and has the benefit of being quick and easy, but it is difficult or impossible to use quantitatively.
Dynamic headspace techniques, in contrast, have been around for years, and have the benefit of being able to produce an aroma profile that is representative of that actually experienced by the consumer. They are also fast and flexible, allowing a range of sample sizes to be run in a relatively short time. The concentration and release step used for dynamic headspace techniques is usually thermal desorption (TD). In this case, the vapours are ‘focussed’ on a sorbent trap, before being released in a narrow band of gas by the application of heat. This process is usually carried out twice, giving massive concentration enhancements that make possible the detection of analytes at the part-per-billion level or below8.
Whichever extraction technique is used, liquid or gas chromatography (LC or GC) is then used to separate the components from each other, followed by analysis. Sensitivity is the key here, as the aroma experienced by the consumer often depends on some of the less abundant components, such as sulphur compounds1.
The human nose is of course pretty sensitive, and can rival analytical techniques for a number of chemicals. The classic example is 1-p-menth-1-ene-8-thiol, which is responsible for fresh juicy smell of grapefruit, and can be detected by humans at levels as low as 0.1 ppt9. This sensitivity is put to good use in GC–olfactometry, which involves exposing the nose to the output of a gas chromatograph10. This has the clear benefit of associating a particular GC peak with a certain smell, but of course it requires a specially trained olfactometrist, which poses limitations for large-scale routine work. In addition, humans can’t do analyte identification or quantitation, or separate co-eluting compounds, and this is where mass spectrometry (MS) comes in.
The combination of GC and MS offers a lot to the cheese analyst, as we’ll see below, but it has taken a while to become a mainstream technique in this field. Part of this is down to cost, and it’s true that it does involve a considerable initial outlay. However, GC–MS is readily automated, so once a method is set up, samples can be processed rapidly and reliably. It’s now even possible to overcome the historic need for rigorous sample prep, by combining it with dynamic headspace sampling, which by definition only extracts the volatiles, leaving the complex sample matrix behind.
One aspect of mass spectrometry that has implications for cheese analysis is the development of time-of-flight (TOF) mass spectrometers designed specifically for GC. Historically, TOF instruments have been viewed as large, expensive and somewhat inaccessible for most laboratories, but these new TOF instruments are much more appealing. This is because they allow the results of a single run to provide all the information needed to comprehensively identify the VOCs even in complex samples, from the main components right down to trace-level chemicals.
[caption id="attachment_29023" align="alignright" width="200" caption="Figure 2: The grated Cheddar sample in the Micro-Chamber/Thermal Extractor used to collect the headspace sample shown in Figure 1. The second chamber shows the lid down, with the sorbent tube in place for collection of the vapours released."][/caption]
To demonstrate what can be achieved using the latest advances in dynamic headspace sampling, thermal desorption, GC and TOF MS, Figure 1 shows the analysis of the headspace of a sample of supermarket mature Cheddar, taken using a micro-chamber sampler11. Here, the cheese was simply grated and placed in a 44 mL chamber, which was then sealed and supplied with a stream of inert gas (Figure 2). Heating the chamber slightly was enough to release the vapours, which were collected on a sorbent trap and fed through a TD–GC system, using a TOF MS for detection.
In Figure 1, each point in the chromatogram is associated with a mass spectrum. In high-speed MS techniques such as TOF, many thousands of full spectra can be obtained per second, and here the data was grouped to give two spectra per second, to maximise the sensitivity. Software was used to process this data to separate chromatographic peaks from baseline anomalies, and to match the resulting spectra against a spectral library of target cheese-aroma compounds, to see which were present. In addition, peaks from compounds not in the target library were matched against comprehensive libraries of chemical compounds.
Almost 70 compounds were detected in a preliminary search (and this was by no means an exhaustive). In addition, a deconvolution algorithm incorporated into the software was able to analyse the changing profiles of individual fragment ions, allowing the identification of co-eluting compounds (Figure 3). Clearly, this is something that would be near-impossible to achieve using the standard approach of matching peaks against the retention times of known compounds.
The principal components in this example are well-documented Cheddar analytes such as 3-hydroxybutan-2-one (#32, aka acetoin), butane-2,3-dione (#10), and fatty acids, including acetic (#41), butanoic (#46), hexanoic (#53) and octanoic (#59) acids. 3-Hydroxybutan-2-one and butane-2,3-dione typically have a pleasant buttery odour12, while the short-chain fatty acids provide vinegary notes that have an increasingly ‘rancid’ character as the chain length rises1. Also relatively abundant was heptan-2-one (#27), variously described as contributing blue cheese, fruity, musty and soapy notes12.
[caption id="attachment_29025" align="alignleft" width="200" caption="Figure 3: The TargetView deconvolution and library-matching software used allows compounds to be detected even when they’re hidden underneath larger components, as demonstrated here for the case of phenylethyl alcohol, which is partly obscured by a peak from dimethyl sulphone."][/caption]
Minor components of the Cheddar headspace included phenylethyl alcohol (#55), which can provide a ‘rosy/floral’ odour to cheese13 – although despite the name of this category, it is usually considered to be undesirable. Hexanal (#18, with a ‘green’ odour12) was also found, as well as two Strecker aldehydes – 2-methylbutanal (#5) and 3-methylbutanal (#6), – which have been reported to be important in the development of a ‘nutty’ flavour in Cheddar14.
Interestingly, one of the components identified – 2,6-diisopropylnaphthalene (#62) – is likely to arise from a non-natural source, as it has been used in the food packaging industry, and also as a plant growth regulator. The presence of such compounds emphasises the potential of this type of method to evaluate foods for the presence of contaminants, as well as odour components.
Overall, it’s the combination of methods – dynamic headspace with TD, GC, the latest TOF MS instruments and post-run software – that makes it a powerful complete solution for identifying the components of cheese aromas. Related applications could include examining the authenticity of cheeses, monitoring the quality of raw materials, understanding the development of aromas during processing and storage, controlling and accelerating the ripening process, as well as identifying undesirable components, such as chemicals from packaging.
Clearly, then, with GC–olfactometry providing the initial information about the aroma contributions from individual components, and dynamic headspace–TD–GC–MS allowing comprehensive routine screening for target compounds and unknowns, the future for investigations of cheese aromas looks very promising.
References
1. P. M. G. Curioni and J. O. Bosset, Key odorants in various cheese types as determined by gas chromatography-olfactometry, International Dairy Journal, 2002, 12: 959–984.
2. A. Subramanian and L. Rodriguez-Saona, Chemical and instrumental approaches to cheese analysis, in: Advances in Food and Nutrition Research, 2010, vol. 59, ch. 5.
3. M. E. Fleming-Jones and R. E. Smith, Volatile organic compounds in foods: A five year study, Journal of Agriculture and Food Chemistry, 2003, 51: 8120–8127.
4. M. Talavera-Bianchi and D. H. Chambers, Simplified lexicon to describe flavor characteristics of Western European cheeses, Journal of Sensory Studies, 2008, 23: 468–484.
5. M. A. Drake et al., Development of a descriptive language for Cheddar cheese, Journal of Food Science, 2001, 66: 1422–1427.
6. K. R. Cadwallader et al., Characteristic aroma components of British farmhouse Cheddar cheese, Journal of Agricultural and Food Chemistry, 2001, 49: 1382–1387.
7. G. Reineccius, Flavour analysis, in: Source book of flavours, Chapman and Hall (New York), 1994, ch. 2.
8. About thermal desorption – A basic introduction, Application Note TDTS 12, Markes International (http://www.markes.com/Downloads/Application-notes.aspx).
9. E. Demole, P. Enggist and G. Ohloff, 1-p-Menthene-8-thiol: A powerful flavor impact constituent of grapefruit juice (Citrus parodisi Macfayden), Helvetica Chimica Acta, 1982, 65: 1785–1794.
10. C. M. Delahunty, G. Eyres and J.-P. Dufour, Gas chromatography-olfactometry, Journal of Separation Science, 2006, 29: 2107–2125.
11. Rapid aroma profiling of cheese using a Micro-Chamber/Thermal Extractor with TD–GC/MS analysis, Application Note TDTS 101, Markes International (http://www.markes.com/Downloads/Application-notes.aspx).
12. T. K. Singh, M. A. Drake and K. R. Cadwallader, Flavor of Cheddar cheese: A chemical and sensory perspective, Comprehensive Reviews in Food Science and Food Safety, 2003, 2: 139–162.
13. M. E. C. Whetstine, K. R. Cadwallader and M. A. Drake, Characterization of aroma compounds responsible for the rosy/floral flavor in Cheddar cheese, Journal of Agricultural and Food Chemistry, 2005, 53: 3126–3132.
14. M. A. Drake et al., Characterization of nutty flavor in Cheddar cheese, Journal of Dairy Science, 2004, 87: 1999–2010.
The author: Dr David Barden, Markes International, Llantrisant, Cardiff, UK
Contact details enquiries@markes.com and enquiries@almsco.com +44 (0)1443 230935 (Markes) and +44 (0)1443 233920 (ALMSCO) http://www.markes.com/ and http://www.almsco.com/