Unravelling the cytoskeleton
22 Dec 2011 by Evoluted New Media
Examining the proteins associated with the cytoskeleton has proved to be a tricky prospect. Here we learn that it is possible to selectively purify this group of important biomolecules and understand some of their complex interactions
[caption id="attachment_25831" align="alignright" width="200" caption="Atomic structure of an actin filament with 13 subunits, based on actin filament model of Ken Holmes. Credit Thomas Splettstoesser"][/caption]
The actin cytoskeleton is a highly dynamic network composed of actin polymers and a large variety of associated proteins. The function of the actin cytoskeleton is to mediate a variety of essential biological functions in all eukaryotic cells, including intra- and extra-cellular movement and structural support1,2. To perform these functions, the organisation of the actin cytoskeleton must be tightly regulated both temporally and spatially. Many proteins associated with the actin cytoskeleton are thus likely targets of signalling pathways that control actin assembly. Actin cytoskeleton assembly is regulated at multiple levels, including the organisation of actin monomers (G-actin) into actin polymers and the super-organisation of actin polymers into a filamentous network (F-actin – the major constituent of microfilaments)3. This super-organisation of actin polymers into a filamentous network is mediated by actin side-binding or cross-linking proteins4-6. The actin cytoskeleton is a dynamic structure that rapidly changes shape and organisation in response to stimuli and cell cycle progression. Therefore, a disruption of its normal regulation may lead to cell transformations and cancer. Transformed cells have been shown to contain less F-actin than untransformed cells and exhibit atypical coordination of F-actin levels throughout the cell cycle7. Orientational distribution of actin filaments within a cell is, therefore, an important determinant of cellular shape and motility.
[caption id="attachment_25824" align="alignleft" width="200" caption="Figure 1. Cytoskeleton enrichment procedure."][/caption]
Focal adhesions and adherent junctions are membrane-associated complexes that serve as nucleation sites for actin filaments and as cross-linkers between the cell exterior, plasma membrane and actin cytoskeleton8. The function of focal adhesions is structural, linking the extracellular matrix (ECM) on the outside of the cell to the actin cytoskeleton on the inside of the cell. They are also sites of signal transduction, initiating signalling pathways in response to adhesion. Focal adhesions consist of integrin-type receptors that are attached to the ECM and are intracellularly associated with protein complexes containing vinculin (universal focal adhesion marker), talin, ?-actinin, paxillin, tensin, zyxin and focal adhesion kinase9,10.
[caption id="attachment_25825" align="alignright" width="200" caption="Figure 2. Western blot of compartmental proteins extracted from HeLa cells. GAPDH (Panel A) is present in the soluble cytoplasmic (lane S) and nuclear (lane N) fractions, and the intermediate filament protein vimentin (Panel B) is present exclusively in the cytoskeletal compartment (lane C)."][/caption]
Studying the proteins that associate with and regulate the actin cytoskeleton has been difficult because of the insolubility of the cytoskeleton in traditional detergents like Triton-X100. Work over the years has shown that many actin regulatory proteins/phospho-proteins upon activation move from the soluble cytoplasmic compartment to the insoluble actin cytoskeleton. The insolubility of these important proteins has made it difficult to study their biochemical changes, such as phosphorylation and nitrosylation, upon binding to the actin cytoskeleton. Here we describe a cytoskeleton purification and enrichment method (ProteoExtract Enrichment and Isolation/Staining Kits, Merck Millipore ) that allows for the selective enrichment of cytoskeleton-associated proteins for detailed protein biochemical analyses. This method provides the means to directly study this important pool of proteins in normal and diseased cytoskeletons, without major disruption to the native cytoskeleton conformation or the native cytoskeleton-protein associations.
We utilised these methods to enrich and either isolate or stain the native cytoskeleton-associated proteins and to minimise interference by soluble cytoplasmic and nuclear proteins. Figure 1 illustrates the method flow chart for the two downstream applications. For biochemical analysis, fractions are collected in a stepwise process, whereas for imaging, fixation of native cytoskeleton on the culture surface is performed. The entire extraction process can be completed in approximately 20 minutes.
[caption id="attachment_25826" align="alignleft" width="200" caption="Figure 3. Confocal fluorescence microscopy of non-treated/enriched and treated/enriched HeLa cells. (A) F-actin was detected using TRITC-conjugated phalloidin, (B) cytosolic protein was detected using GAPDH antibody as a cytosolic control and a FITC-conjugated secondary, (C) nuclear counterstaining was revealed with DAPI and all images were overlaid. Soluble cytosolic protein fraction (GAPDH) was successfully removed after enrichment."][/caption]
Western blot analysis indicated that GAPDH is present in the cytoplasmic and nuclear fractions11, whereas the intermediate filament protein vimentin is present exclusively in the cytoskeletal compartment (Figure 2). The ability to detect and study the low abundance cytoskeleton-associated proteins is greatly increased with this cytoskeleton enrichment and isolation method.
The cytoskeleton enrichment and staining method enhances sensitivity of detection of focal adhesion and cytoskeletal proteins by removing soluble cytoplasmic and nuclear proteinsGAPDH is a cytosolic marker which was completely removed by the enrichment procedure (Figure 3, panel B, treated cells). Vimentin represented as an intermediate filament protein was largely retained after the enrichment (Figure 4, panel B, treated cells). Vinculin, an integral component of focal adhesion plaques, is involved in linkage of integrin adhesion molecules to the actin cytoskeleton. Conventional whole cell staining (Figure 5, panel B, non-treated cells) results in some focal adhesions detected on a diffuse background. With the ProteoExtract Cytoskeleton Enrichment kit, the background staining due to soluble cytosolic pool of vinculin was significantly reduced, resulting in sensitive detection of insoluble vinculin solely at focal adhesions (Figure 5, panel B, treated cells). In addition, distribution of ?-catenin, which anchors the actin cytoskeleton to cell-cell junctions, is restricted to cell-cell junctions (Figure 6, panel B, treated cells), as compared to the combination of diffuse and membrane-associated staining in non-treated whole cells (Figure 6, panel B, non-treated cells). Thus, this method for extraction of soluble proteins and enrichment of the insoluble cytoskeleton provides the means for more sensitive analysis of the important pool of proteins in cytoskeleton structure.
- Methodology
[caption id="attachment_25828" align="alignright" width="200" caption="Figure 4. Confocal fluorescence microscopy of non-treated/enriched and treated/enriched HeLa cells. (A) F-actin was detected using TRITC-conjugated phalloidin, (B) cytoskeletal protein was detected using vimentin and a FITC-conjugated secondary, (C) nuclear counterstaining was revealed with DAPI and all images were overlaid. Insoluble cytoskeletal protein fraction (vimentin) was successfully retained after enrichment."]
[/caption]
Human HeLa cells were grown in DMEM complete medium in 100mm culture dish until 80-90% confluence. The cells were gently washed twice with 2 mL of cold 1x DPBS. Cold 1x cellular extraction buffer (0.25 mL per plate) was added to the cells and incubated for 1.5 minutes on ice. Buffer was collected and labelled as soluble compartment (S). 0.5 mL 1x cytoskeleton wash buffer was added to the cells and then pooled with the soluble compartment. Nuclear extraction buffer (0.25 mL per plate) was added to the cells and incubated on ice for 10 minutes. This fraction was collected and labelled as nuclear compartment (N). Cells were washed twice with 2 mL of 1x cytoskeleton wash buffer.To isolate the enriched cytoskeleton compartment, 0.25 mL of cytoskeleton, solubilisation buffer was added to the cells following the cytoskeleton wash buffer step and pipetted up and down for maximum solubilisation. This fraction was collected and labelled as cytoskeleton compartment (C). Protein concentrations of all collected compartments were determined by A280 and stored at -80°C until further application as described below.To visualise the enriched native cytoskeleton, human HeLa cells were grown in DMEM complete medium in 8-well glass chamber slides until 80-90% confluence. Extraction of soluble and nuclear compartments was performed as above, except that these fractions were discarded by aspirating at each step. The adherent insoluble cytoskeleton remaining attached to the chamber slide was fixed by addition of 0.25 mL 4% paraformaldehyde to each well following the last cytoskeleton wash buffer step to fix the cells. After a 30-minute incubation at room temperature, the cells were washed with 0.25 mL of 1x DPBS per well.After fixing the native cytoskeleton, the cells were washed twice with 0.5 mL 1x blocking/permeabilisation buffer and incubated with 0.25 mL/well diluted primary antibody in 1x blocking/permeabilisation buffer for one hour at room temperature. Washes and incubation with a dye-conjugated secondary antibody, TRITC-conjugated phalloidin (1:100) and DAPI (1:200), each provided in the staining kit, were performed to stain the actin cytoskeleton and remaining cell nucleus. Cover slips were mounted on slides with mounting fluid and visualised with a fluorescence microscope.Following the enrichment of the cytoskeleton, collected cell compartments (S, N and C) were subjected to SDS-PAGE gel and transferred to a PVDF membrane. A standard western blot procedure was applied, with GAPDH and vimentin antibodies (provided in the kit) to detect cytosolic and intermediate filament proteins, respectively.
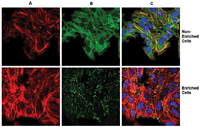
References
1. Chen C.S. et al, 2003. Cell shape provides global control of focal adhesion assembly. Biochemical and Biophysical Research Communications, Vol. 307 (No. 2) 355–61.
2. Frixione E., 2000. Recurring views on the structure and function of the cytoskeleton: A 300-Year Epic. Cell motility and the cytoskeleton, Vol. 46 (No. 2) 73–94.
3. Bretscher A. et al., 1994. What are the basic functions of microfilaments? Insights from studies in budding yeasts. Journal of Cell Biology, Vol 126 (No. 4) 821-825.
4. Dubreuil R.R., 1991. Structure and evolution of the actin crosslinking proteins. BioEssays, Vol. 13 (No. 5) 219-226.
5. Matsudaira P., 1991. Modular organization of actin crosslinking proteins. Trends in Biochemical Sciences, Vol. 16 87-92.
[caption id="attachment_25833" align="alignright" width="200" caption="Figure 6. Confocal fluorescence microscopy of non-treated/enriched and treated/enriched HeLa cells. (A) F-actin was detected using TRITC-conjugated phalloidin, (B) cell junctions were detected using beta-catenin antibody and a FITC-conjugated secondary, (C) nuclear counterstaining was revealed with DAPI and all images were overlaid. Background due to soluble cytosolic fraction was significantly reduced after enrichment, resulting in clear detection of insoluble, low-abundance actin-associated proteins (beta-catenin)."][/caption]
6. Matsudaira P., 1994. Actin crosslinking proteins at the leading edge. Seminars in Cell Biology, Vol. 5 (No. 4) 165-174.
7. Rao J.Y. et al., 1990. Cellular F-actin levels as a marker for cellular transformation: relationship to cell division and differentiation. Cancer Research, Vol. 50 2215-2220.
8. Yamada K.M., Geiger B., 1997. Molecular interactions in cell adhesion complexes. Current Opinion in Cell Biology, Vol. 9 (No. 1) 76-85.
9. Burridge K. et al. 1990. Actin-membrane interaction in focal adhesions. Cell Differentiation and Development, Vol. 32 (No. 3) 337-342.
10. Turner C.E., Burridge K., 1991. Transmembrane molecular assemblies in extra-cellular matrix interactions. Current Opinion in Cell Biology, Vol 3 (No. 5) 849-853.
11. Tristan C. et al,.2011. The diverse functions of GAPDH : views from different subcellular compartments. Cellular Signaling, Vol 23 (No. 2): 317-323.
Authors: Haizhen Liu, Karyn Huryn, Jun Ma and Luke Armstrong