Beyond the code
22 Jul 2019
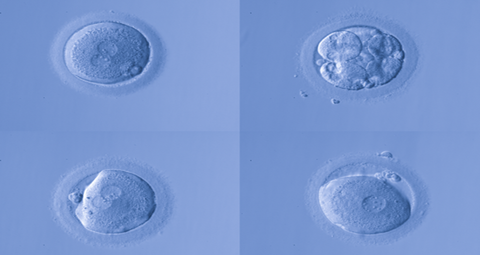
Genomics has been revolutionary – but have we forgotten cell biology in the push for an ever more reductive understanding of health and disease? Professor John S Torday thinks the key, not just to managing disease, but to understanding evolution itself lay not in the genes but in a focus on cellular interactions...
In many ways, the modern discipline of biology began with Linnaeus’s use of binomial nomenclature nearly 300 years ago. Subsequent progress has been achieved through reductionist science, culminating in publication of The Human Genome.
Since that epic achievement there has been no medical advancement using this highly coveted set of data. Instead, I’d argue we find ourselves in something of a crisis in biomedical research. We could attribute this to the way science is taught, lack of funding, superannuated post-doctoral training, but is that right? Could it be possible that we have been reasoning after the fact about the origin and causation of life itself?
The discovery of the DNA double helix was arguably the biggest breakthrough in biology. It essentially changed our way of thinking about life, convincing us that our fate is due to “code”. Yet the process of embryologic development, which is the only mechanism known to generate form and function, is determined by cell-cell interactions mediated by soluble growth factors.
We do not have a central theory of biology, akin to the central theory of physics and chemistry
That phenomenon was discovered more than 40 years ago. It provided a way of understanding embryo development for the first time since German embryologist Hans Spemann identified the “organiser” for developmental processes but had no way of testing that hypothesis further. Nor could the idea that “ontogeny recapitulates phylogeny” be experimentally tested, so the evolutionists turned to genetics as the basis for scientifically advancing evolution theory. From that time to this, I’d say cell biology has been excluded from evolutionary biology, leaving a void between evolution theory, biology and medicine.
Genetics gave rise to the modern theory of evolution, allowing for quantitative descriptions rather than mere metaphors and similes. Much has been written about evolution in the interim, beginning with Darwin’s On the Origin of Species, Waddington’s The Strategy of the Genes, Gould’s Ontogeny and Phylogeny and Dawkins’ The Blind Watchmaker, among others. Yet there is literally no experimental evidence for the mechanistic evolution of form and function – such as the phylogenetic change from fish to man for example – that relates to causation.
But there are, of course, lots of inferences from observation – e.g., the fossil record – that tell you evolution is real. They just do not tell you anything about how or why it occurred – so the problem as I see it is that the wrong inference was made about causation. No wonder there is debate about creationism/intelligent design versus evolution theory. One belief is just as acceptable as another, whereas scientific evidence would largely put an end to such discussions, just as the data provided by Copernicus, Keppler and Galileo did for the Sun as the centre of the Solar System, or Pasteur’s experimental evidence refuting spontaneous generation, for example.
Biology remains descriptive
We do not have a central theory of biology, akin to the central theory of physics and chemistry – starting with the Big-Bang as the origin of the laws of nature. There has been no set of first principles for biology.
Consequently, the lung has long been thought to have evolved from the gills of fish since both organs are used for gas exchange. However, when seen from the perspective of their cellular and molecular compositions, the lung is a homologue of the swim bladder, the organ that allows the fish to adapt for buoyancy. At first this would seem counterintuitive, but once the different cellular and molecular components are seen in light of the underlying mechanisms supporting gas exchange, the commonalities become clear. And as I will discuss later in the context of human development, both the swim bladder and the lung are reliant on the production of surfactant, a soapy material that keeps both the chamber for gas exchange in the bladder and the alveoli of the lung functional.
As a result of the descriptive, non-mechanistic status of biology, it remains a “soft” science, unable to predict how long we will live, or whether we will contract a specific illness. Medicine can offer probabilities based on epidemiologic correlations and associations, but not certainties based on molecular genetics, unlike the effect of gravity, or the product of a chemical reaction. In terms of medical advancements, the descriptive approach produces huge bodies of knowledge, but how much of it is useful for medical development? In my view it moves us away from where medicine needs to go. Indeed, the probabilities are largely based on epidemiology instead of on molecular genetics.
A novel approach
Some 50 years ago it was discovered that patterns of embryologic development were formed through cell-cell interactions mediated by soluble proteins termed growth factors. My laboratory stumbled on to this property of cells in the mid-1970s in search of the mechanism by which the simple hormone, cortisol, stimulated the complex physiologic development of the fetal lung in the womb. This serendipitous finding was significant because a functioning lung is the rate-limiting step in the transition of the offspring from the mother to the hospital delivery room. President John F. Kennedy’s baby died of hyaline membrane disease in 1962, a disease of lung surfactant deficiency, so it fortunately or unfortunately made the public aware of this developmental disease.
We were investigating the effects of cortisol on developing lung cells in culture, showing that we could accelerate the production of lung surfactant, the missing factor during the process of lung development. However, to our surprise, the effect was not on the epithelial lining cells of the alveoli where the surfactant is produced, but on the connective tissue cells lying directly underneath the epithelial cells. The connective tissue cells were found to stimulate the production of surfactant by means of what was called fibroblast pneumocyte factor, a small protein that stimulated the epithelial lining cells to produce surfactant.
As a result of this discovery, cortisol is now used worldwide clinically in women threatening to give birth prematurely, saving the lives of millions of newborns annually.
In contrast to this systematic approach to the cellular mechanisms involved, contemporary biomedical research transcends the biologic properties of disease, and can all too often reduce it to DNA nucleotide sequences, failing to discern the causal cellular relationships that would otherwise provide means of diagnosing and treating diseases safely and effectively.
The epigenetics of development
Subsequently, many such maturational mechanisms that culminate in homeostasis, i.e. biologic equilibrium, were found to determine pattern formation in the developing embryo, mediated by soluble growth factors. Since all diseases are, in essence, due to loss of homeostasis, such embryologic mechanisms provide insight to how and why diseases occur.
The sensitivity and specificity of the effects of such growth factors were mediated by receptors that regulated the growth and differentiation of neighboring cells of different embryonic origins. These processes are the only way presently known for the formation of structure and function in biology. And since they affect cell growth and differentiation through cell-cell interactions, their mechanism of action is epigenetic.
As such, growth factor mediated cell-cell interactions offer the opportunity to reverse-engineer the evolution of physiologic traits that generate phylogenetic changes, otherwise known as evolution. With that in mind, the evolution of the lung was traced all the way back in time to the unicell, when cholesterol appeared in the cell membrane. The reverse engineering of development and phylogeny based on cell-cell communication mechanisms has never been applied to evolution before, because Darwinists believe that evolution is solely due to random genetic mutations. Therefore there would be no way of determining causation, but it seems to me they are misguided in making that assumption.
Konrad Bloch had discovered the cholesterol synthetic pathway, hypothesizing that there had to have been enough oxygen in the atmosphere to do so, given that it takes 11 atoms of oxygen to synthesize one molecule of cholesterol. Cholesterol rendered the cell membrane thinner, facilitating gas-exchange, metabolism and locomotion, the three key traits for vertebrate evolution. By turning the cell-cell interactions of development around 180 degrees, the phylogenetic evolution of the lung could be seen from its origins forward mechanistically for the first time.
To reiterate, developmental biology is the basis for generating biologic form and function, and phylogeny is the change in structure and function over the course of evolution. Therefore, development underpins the mechanisms of phylogeny. Conventionally, this is referred to as evolutionary developmental biology, or evo-devo. However, as it is practiced by strict Darwinists, it is only described, not dealt with longitudinally based on cell-cell communication mechanisms, since it is believed to strictly be due to random genetic mutations. In contrast to that, by systematically focusing on the developmental and phylogenetic mechanism for increasing the surface area-to-blood volume ratio of the alveolus to facilitate gas exchange, the step-wise changes in the cellular composition of the latter mediated by soluble growth factors and their receptors can now be seen to determine such changes evolutionarily.
Cell-cell interactions
Such a step-wise process, based on experimental evidence, offer a novel way of thinking about the overall mechanism of physiologic evolution. Conventionally, physiologic evolution is described as a series of interrelated events without any underlying cellular-molecular mechanisms, again, because of the central belief that Darwinian evolution is due to random genetic mutations. To test the predictive power of this approach, documented specific gene duplications and phenotypic structural-functional changes associated with the water-land transition were exploited to determine the effect of cell-cell signaling on lung evolution. Given that the process of lung evolution occurred in a step-wise cellular manner, it was reasoned that there would have been episodes of hypoxic low levels of oxygen due to the inability of the evolving lung to provide adequate amounts of oxygen for metabolism. That would have caused physiologic stress, hypoxia being the most potent physiologic stressor, stimulating the pituitary-adrenal axis to produce adrenaline; adrenaline would have alleviated the oxygenation constraint on the alveoli by physiologically stimulating the production of lung surfactant, making the alveoli more distensible, acutely increasing oxygenation. Subsequently, more alveoli formed in response to the distension of the lung, consistent with the development and phylogeny of gas exchange in vertebrates.
This perspective on evolution, based on the historic ways in which physiologic traits have responded to environmental change, provides an integrated view for the underpinnings of health and disease as a continuum rather than as mutually exclusive binaries. This is a radical departure from the way in which medicine is currently practiced because it offers the opportunity to detect the loss of homeostasis long before the individual becomes a patient.
Such early detection would save billions of dollars in healthcare costs annually, not to mention the relief from the pain and suffering from the morbidity and mortality caused by having to wait until the patient becomes symptomatic. Moreover, by specifically identifying the cellular-molecular site of the problem, the disease can be targeted more specifically.
And the central role of homeostasis acting to generate, maintain and regain cell-cell signaling has provided a holistic way of thinking about biology and medicine.
A path beyond genetics: Epigenetics and disease etiology
As mentioned, embryologic development and homeostasis are due to the epigenetic effects of cell-cell interactions. There are numerous examples of epigenetic mechanisms for disease. For example, my laboratory studies the epigenetic mechanism underlying the well-recognised effect of cigarette smoke on childhood asthma. Several years ago it was discovered that the biggest epidemiologic signal for this phenomenon was not whether your parents smoked, but whether your grandmother smoked, suggesting epigenetic inheritance between multiple generations.
To study this hypothetical mechanism, we chose to use nicotine as a proxy for cigarette smoke due to the complex composition of smoke, containing some 3,000 bioactive compounds. We treated pregnant rats with nicotine during pregnancy to mimic the effect of smoking on the developing early embryo and discovered that the offspring developed the asthma phenotype molecularly, biochemically, histologically and functionally. When the asthmatic offspring were crossbred, we found that the nicotine effect persisted for several generations.
Transgenerational psychological depression is another example. It has been shown that if a mother rat is physiologically stressed during pregnancy, her circulating cortisol levels increase and cross the placenta into the developing embryo. The elevated cortisol level re-sets its hypothalamic-pituitary-adrenal axis, resulting in chronic hypercortisolism in the offspring, which is known to cause depression.
And the well-recognised obesity epidemic has been attributed to such epigenetic effects as well. Maternal obesity leads to obesity in the offspring for multiple generations, likely due to the excessive use of corn syrup in the American diet creating an overabundance of inexpensive nutrients. Transgenerational effects of excess nutrients on childhood obesity have been demonstrated experimentally.
Implications of epigenetic inheritance
There is a common thread between altered fetal development, evolution and epigenetic inheritance mediated by cell-cell communication. This perspective is radically different from our current way of thinking about health and disease. Tremendous resources and effort have been applied to the genetic origins of disease, yet the evidence is that diseases inherited in such a way are few in number, representing less than 5% of inherited diseases. On the contrary, evidence for the direct epigenetic effects of the environment on development and disease is rapidly accumulating. When combined, cellular evolution and epigenetic inheritance provide a continuum from health to disease, rather than thinking of health and disease as mutually exclusive binary states of being.
Furthermore, understanding the interrelationships between health and disease at the cellular-molecular level provides insights to the evolutionarily physiologic interventions that will restore homeostasis, just like nature would do, given enough time and resources.
By viewing phylogeny as a series of step-wise cellular-molecular changes to sequentially establish and re-establish homeostasis, whether the patient is healthy or ill could be assessed using medical imaging techniques for the overall status of intermediate signaling mechanisms. Clearly, there are those of us who may experience loss of homeostasis but are genetically able to mount an effective physiologic response and heal ourselves. Such patients could be actively surveilled as is now done for those with prostate cancer. In contrast to that, if the homeostatic loss persists and becomes more pervasive the physician could intervene using specific pharmacologic means to re-establish the cellular-molecular balance.
Technologically, this view of health and disease lends itself to novel diagnoses and treatments based on imaging for the detection of lost homeostasis and targeted therapy based on such evidence. Imagine a procedure by which a “tagging” method based on magnetic force would be used to detect lost homeostasis body-wide. Based on the localisation of the tag, the magnet in the magnetic resonance imaging device could theoretically be used to both detect and correct the detected loss of homeostatic control. That would truly represent evolutionary and preventive medicine.
Author: Professor John S. Torday is Professor and Director of The Henry L. Guenther Laboratory for Cellular-Molecular Biology at Harbor-UCLA and a Professor of pediatrics at the Los Angeles Bio Medical Research Institute. He is also Professor in Residence for Pediatrics, Obstetrics and Gynecology, and Evolutionary Medicine at UCLA