Time to be selective
15 Dec 2019
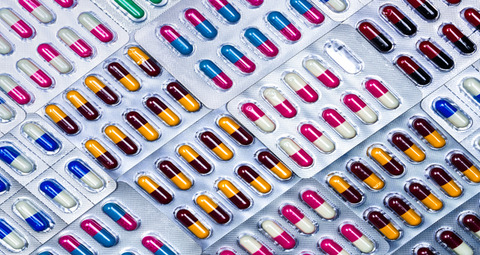
As the AMR crisis looms large, we would do well to look beyond classic drug targets says Dr Thomas Böttcher. Concentrating on bacterial signalling molecules could hold the keys, not only to a new generation of selective microbials but also to managing our own microbiome…
Antibiotic resistant pathogens are on the rise and pose a major challenge for human health.
Even pathogens that have been long thought to be defeated such as Neisseria gonorrhoeae – the causative agent of the sexually transmitted disease gonorrhoea – have infamously made their comeback due to a lack of treatment options for resistant strains. The World Health Organization (WHO) keeps constantly updating its priority list of pathogens that currently cites 12 families of bacteria which, due to their resistance profile, require urgent need for action.
Moreover, the discovery of new antibiotics has been declining and the majority of marked approved antibiotic drugs belong to a handful of different compound classes that are matched by an almost equally low diversity of cellular targets. Resistance mechanisms, such as efflux pumps, thus frequently affect a large subset of available drugs and target mutations render bacteria resistant to multiple mechanistically related drugs leading to the rapid evolution of multidrug resistant pathogens. These strains are major risks factors for immune compromised patients and often present life threatening or fatal consequences.
Disrupting quorum sensing signaling could allow the disarmament of pathogenic bacteria and may, in the future, present an alternative treatment option to antibiotics
Increasingly, however, multidrug resistant pathogens also infect previously healthy people in the community and the prognosis are alarming. By 2050, more people may be killed by antibiotic resistant microbes than by cancer. This unfolding crisis has been sparked by a variety of factors. Leading problems are the continuing overuse of antibiotics mainly in industrial livestock farming and malpractices of hygiene measurements in intensive care units that contribute to the development and spread of resistance mechanisms. Horizontal gene transfer ultimately allows the transmission of resistance genes across genus and species boundaries. This, in combination with the low diversity of drug classes and cellular targets, is the reason why some strains are close to becoming untreatable. In addition to rethinking antibiotic use and applications, new antibiotics are urgently needed.
Friendly fire
Yet, the majority of microbes that are affected by antibiotics are not pathogens but commensals colonizing, for example, our skin, soft tissues, the respiratory system or the gastrointestinal tract. The human body is a true microcosm and hosts even more microbes than human cells – estimates suggest about 40 trillion microbial cells for an average healthy adult.
The majority of these microbes live peacefully with us – at least most of the time – and some of them are essential to human health. This ecosystem, the human microbiome, is fragile and a shift in equilibrium in its composition or biodiversity can have serious consequences. Many human diseases can be understood as a result of dysbiosis – a pathological imbalance or perturbation of the microbiome. These include inflammatory bowel disease, colitis and oral infections (periodontitis) but also obesity, cancer and autoimmune diseases have been shown to have links with microbial dysbiosis.
Research of the past decades has demonstrated the importance of interactions between the gastrointestinal tract and the central nervous system, the so-called gut-brain axis. It is possible therefore that gut microbiota play a central role in neurodegenerative disorders such as Alzheimer’s and Parkinson’s disease as well as psychiatric illnesses –both of which have been associated with altered microbial colonisation.
The importance of an intact microbiome is highlighted by the diversity of effects that its disruption has on human health. However, even short-term antibiotic treatments may irreversibly alter the fragile balance within the human microbiome. This is problematic especially since most antibiotics have broad spectrum activity against multiple microbial species and often even inhibit a wide range of gram-positive as well as gram-negative species. Thus, pathogenic bacteria are targeted alongside beneficial commensal species.
Clearing entire fractions of the microbiome creates free niches which can be occupied by non-susceptible species or antibiotic resistant strains. But how can we treat microbial infections without impairing functional microbiota in the human body?
Quorum sensing breakthrough
The answer could be more selective antibiotics that only inhibit growth of a defined, narrow range, of pathogens or even a single species. Recent work by my team at the University of Konstanz has demonstrated that highly species-selective antibiotics are already now within reach and can be found by synthetically exploiting the repertoire of natural products produced by microbes.
The human pathogen Pseudomonas aeruginosa produces a large diversity of metabolites of the quinolone-class. Some of them have antibiotic properties and allow the bacteria to defend its niche against competing bacteria such as Staphylococcus aureus. This competition of pathogens within the human body inspired us to explore further these natural products. Other quinolones, like the compounds HHQ and PQS, are so called quorum sensing signals which the bacteria use to coordinate their behavior in dependence of population density. Cells produce and excrete the signal molecules but also can sense their presence by a receptor protein. When the signal binds to the receptor, the transcription of certain genes is enhanced including those encoding the biosynthetic machinery for signal production. This leads to a positive feedback loop that allows the cells to measure how many of their kind around.
Along with signal production, genes are also activated that encode for virulence factors like toxins that help the bacteria to infect their human host. Coordinating their virulence factor production in relation to population density has important advantages: being silent at low cell densities may help to hide from the immune system, while at high densities all cells secreting virulence factors at the same time allows a coordinated attack and supports the infection process.
Disrupting quorum sensing signaling could allow the disarmament of pathogenic bacteria and may, in the future, present an alternative treatment option to antibiotics. This possibility has also been explored for the quinolone signals of the important pathogen P. aeruginosa. In more recent work my team discovered that the quorum sensing signal PQS has roles beyond signaling. When we tested the compound against various human commensals and pathogens, they noticed highly selective antibacterial properties against another species: Moraxella catarrhalis. This pathogen resides in the respiratory tract and frequently causes middle ear infections in children as well as infections in patients with chronic obstructive pulmonary disease (COPD). While PQS inhibited growth of Moraxella catarrhalis, other species of the human microbiome remained entirely unaffected. The selectivity was outstanding, yet the activity of the natural product was rather low.
In order to build on this, we applied synthetic chemistry and engineered the compounds core structure. After a few optimisation steps a new antibiotic compound was born that was still as selective as the natural product but with highly improved activity in the range of commercially available antibiotics. A key difference from these commercial antibiotics, however, was that the engineered compound only targeted the pathogen but not commensal strains even of closely related species.
Further development of this approach in order to create drugs for human use has a long way to go and, like any drug development, will be fraught with a high risk of failure. However, the results demonstrate that promising biological activities are easily overlooked and can be found in relatively simple bacterial metabolites that have been known for decades.
Exploiting these activities by chemical engineering of natural product scaffolds can yield new antibiotics with unprecedented species-selectivity. In the future, such compounds could allow customised treatment of bacterial infections and open an era of microbiome engineering with precision tools.
Further reading:
Szamosvári, T. Schuhmacher, C. Hauck, T. Böttcher (2019) A thiochromenone antibiotic derived from Pseudomonas quinolone signal selectively targets the Gram-negative pathogen Moraxella catarrhalis. Chem. Sci. 10: 6624-6628.
Prothiwa, F. Englmaier, T. Böttcher (2018) Competitive live-cell profiling strategy for discovering inhibitors of the quinolone biosynthesis of Pseudomonas aeruginosa. J. Am. Chem. Soc. 140 (43): 14019–14023.
Szamosvári, S. Rütschlin, T. Böttcher (2018) From pirates and killers: Does metabolite diversity drive bacterial competition? Org. Biomol. Chem. 16: 2814-2819.
Szamosvári, T. Böttcher (2017) An unsaturated quinolone N-oxide of Pseudomonas aeruginosa modulates growth and virulence of Staphylococcus aureus. Angewandte Chemie Int. Ed. 56(25): 7271-7275.
Author:
Dr Thomas Böttcher, research group leader, department of chemistry, University of Konstanz, Germany