Catching the elusive neutrino
16 Jan 2020
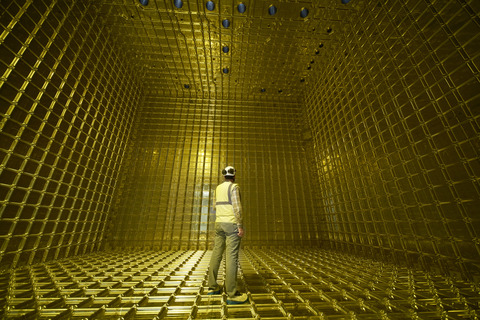
From seeing a blackhole form to understanding antimatter… neutrinos could hold the keys to them all – however they are frustratingly difficult to detect. Here, Dr John Marshall tells us about a new international effort to catch one in the act…
The DUNE experiment sounds incredibly ambitious… tell us a bit about it
The Deep Underground Neutrino Experiment (DUNE) will perform precision studies of the elusive subatomic particles known as neutrinos. We believe that neutrinos are one of the most abundant particles in the universe, but they interact very rarely: trillions of them pass harmlessly through our bodies every second, yet we know relatively little about them. DUNE will study an intense beam of neutrinos and aims to answer fundamental questions about the nature of matter and the evolution of the universe.
DUNE will use the world’s most powerful neutrino beam, manufactured at the Long-Baseline Neutrino Facility at Fermilab in Illinois, USA. This intense beam of neutrinos will be directed 800 miles through the Earth to the huge DUNE detectors located in the Sanford Underground Research Facility in South Dakota. These cutting-edge detectors will be Liquid-Argon Time-Projection Chambers (LArTPCs), which will provide “photograph-quality” images of the charged particles that emerge when one of the neutrinos interacts inside the detector.
A small detector located at Fermilab will ensure that the neutrino beam is well understood near its source. DUNE scientists will be looking for changes in the composition of the neutrino beam during the 800 mile journey to South Dakota. This international project brings together over one thousand scientists from more than 30 countries, hoping to unravel the mysteries of neutrinos.
So, it is clearly a multinational effort – what role do you have in that?
One of the key features of DUNE is its use of cutting-edge LArTPC detectors to record neutrino interactions. We can’t see the neutrinos directly, but we can search for the charged particles that emerge when a neutrino interacts with, for instance, an Argon nucleus in the detector. The LArTPC detectors provide extraordinarily-detailed images of the trajectories of these charged particles.
The images reveal the charged particle trajectories, with spatial resolutions of the order of millimetres. Examining these images, the human eye/brain can usually pick out a well-defined interaction vertex (the point from which particles emerge) and trace the paths of the different particles, identifying whether they go on to interact themselves or decay.
Our role is to examine the images of neutrino interactions and provide an automated, algorithmic “reconstruction” of what happened in the interaction. We’re really mining the LArTPC images for information about the interaction and using computers to build up a picture of what happened in the detector, in the same way as the human brain tries to when presented with the pictures.
It will be an amazing achievement to record fine-granularity pictures of neutrino interactions at DUNE, but we need to extract the information from these pictures and provide a physics-level interpretation of the interactions, in order to meet the ambitious physics goals of DUNE.
Clearly high-end particle physics is very dependent on the technological breakthroughs of instrumentation, but how big a role does the data handling end of things play?
It’s an enormous challenge to handle the data produced by sophisticated detectors with many thousands of readout channels. DUNE requires expertise in all areas, including data acquisition, data storage and management, and all the steps to process or analyse the data. Sophisticated simulations of our detectors are also required in order to help develop software and analyses.
At Warwick, we focus on event reconstruction: the inputs are the images from a LArTPC, showing the charged particles that emerged in the detector. We first need to remove any noise or detector effects, and then sparsify the images, leaving just paths of “hits” along particle trajectories. We then need sophisticated pattern-recognition approaches to identify and characterise the different particles in each interaction. The neutrino interaction images can be extremely complex, with many overlapping particles and a complex mix of track-like and shower-like topologies.
We’re developing a multi-algorithm approach to the pattern recognition and use over one hundred algorithms, backed by a software package called Pandora, to gradually build up a picture of events. The first algorithms can be rather simple, for instance clustering together parts of the image that unambiguously represent single particle trajectories. Later algorithms can be more sophisticated, as they try to collect together all the hits from individual particles and avoid mixing together those hits that were truly from different particles.
We’re also increasingly using developments from the field of computer vision. For instance, we’re using convolutional neural networks (commonly used to classify photographs) in order to identify neutrino interaction vertex positions. Collectively, our algorithms provide a reconstruction that can cope with the diverse and complex event topologies of neutrino interactions.
This is huge project then… will it be worth it? What can we learn from neutrinos?
Neutrinos are all around us, but they typically pass through matter without leaving a trace. We therefore know frustratingly little about neutrinos, but they may provide the key to answering some of the most fundamental questions about our universe. By producing our own beam of neutrinos, and their antiparticles (antineutrinos), and studying them with cutting-edge detectors, DUNE hopes to learn much more.
We believe that the Big Bang should have created equal amounts of matter and antimatter, yet we clearly live in a matter-dominated universe. One of the great outstanding questions in fundamental physics is why we see this matter-antimatter asymmetry and neutrinos could hold the key. Subtle differences in the behaviour of neutrinos and antineutrinos could help explain this asymmetry.
The DUNE detectors could record a burst of about ten thousand neutrinos from a core-collapse supernova in our galaxy, should one occur during detector operations. This would provide an amazing opportunity to “see” neutron star formation or black hole formation in real time. It’s not just about the neutrinos, either, and DUNE’s LArTPC detectors will search for signals of proton decay, which could help formulate a grand unified theory of the fundamental interactions.
Could the pattern recognition technologies that you develop be useful in other areas of physics – or even wider, in other disciplines altogether?
Particle physics has traditionally pushed the boundaries of knowledge and technology. The neutrino event topologies we expect to see at DUNE are so complex and diverse that we are having to bring a fresh perspective to pattern recognition in particle physics. Our approach of breaking up the pattern recognition into many smaller steps is certainly one that could be employed in different use cases, albeit with some significant effort required to tune the performance for each case. Pattern-recognition problems are ubiquitous in the sciences and in industry, and anywhere there’s images that are extremely diverse, then a multi-algorithm approach can bring together different algorithms (with different inherent levels of sophistication) to tackle different aspects of the problem and, collectively, provide a robust automated solution.
Dr John Marshall is an Assistant Professor at the University of Warwick. His research team is developing software algorithms capable of automatically reconstructing neutrino interactions in the DUNE detectors.