What’s your poison?
11 May 2020 by Sarah Lawton
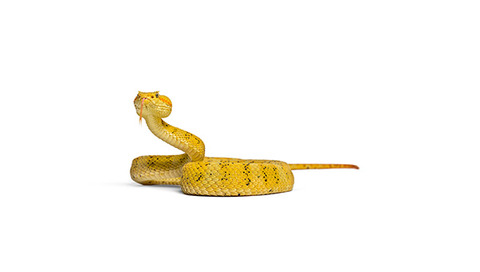
Why are so many drugs derived from natural sources on the market to treat human disease? Venom-master Steve Trim explains how evolution can give us a Pharmaceutical short-cut.
There are many clues in nature as to pharmacological evolution long before the hominid branch of the phylogenetic tree started to sprout. The term ‘evolutionary arms race’ eloquently describes many situations such as the development of antibacterial compounds by fungi and soil bacteria. These organisms are fighting a tough battle for space and resources with millions of competing organisms all the time. Thus, as soon as an organism starts to produce a compound that slows the growth of a competitor it develops a clear advantage in terms of natural selection and therefore this trait is retained and developed.
The evolutionary reason why these venoms have potent effects on humans, is not because the snakes like to eat unsuspecting backpackers, but because the target is conserved in the mammalian branches of the phylogenetic tree.
Steve Trim, Venomtech
Homo sapiens, as a species of opportunistic tool users, discovered such compounds in the late 1940’s and developed them for their own competitive advantage over pathogenic microorganisms. This situation is relatively straight forward – it is clear why the compound has evolved, and it has nothing to do with us, we just discovered it, then utilised it. The antibiotics were actually better for the fact that their evolution was totally independent from ours. However, we are all very familiar with the current situation where pathogenic bacteria are gaining back territory through antibiotic resistance mechanisms and new viruses such as SARS CoV2, having a devastating effect on our population. Viruses have always been challenging to defeat and our best success has been with vaccines rather than treatments. With bacteria the evidence is clear that anthropogenic factors have definitely enhanced the opportunity for pathogenic microbes to evolve resistance mechanisms. With viruses we are still a long way from knowing the all potential viruses that could cause further challenges to ourselves and our food organisms.
Many recent publications and work in progress have identified that venomous animals are also in an arms race with microbes living in their venom glands. Many researchers in this unique area have gotten together to form The Initiative for Venom Associated Microbes and Parasites (iVAMP) to understand the biology of the venom microbiome. Thus not only does this open the door for revised treatment strategies for envenomation patients, but it also gives a clue as to why venoms contain therapeutically useful antimicrobial compounds.
Human targets?
So with this in mind, why are there many venom derived drugs in the clinic that hit human targets and deliver therapeutic benefit? The story starts with the first venom inspired therapeutic which was first in class for a new mechanism to treat the life-threatening problem of hypertension. Captopril was the first ever anti-hypertensive and inhibits the, now well known, Angiotensin Converting Enzyme (ACE). Captopril is a small molecule that mimics the action of bradykinin potentiating peptides found in many viper venoms including the Fer-De-Lance, Bothrops asper.
These peptides inhibit conversion of Angiotensin I to active Angiotensin II (vasoconstrictor) and, as the name suggests, potentiates bradykinin action by inhibiting its degradation. This mechanism was discovered in the late 1960’s and the development of drugs targeting this mechanism has saved millions of people’s lives through treating hypertension. The evolutionary reason why these venoms have potent effects on humans, is not because the snakes like to eat unsuspecting backpackers, but because the target is conserved in the mammalian branches of the phylogenetic tree.
As cryptic ground dwelling ambush predators, the adult lance heads often prey on rodents but will also tackle anything within striking range. Rat (Rattus norvegicus) and mouse (Mus musculus) ACE genes are 80% identical to the human gene, thus it is clearly conserved. The snake’s venom incapacitates small prey through haemodynamic collapse driven by catastrophic hypotension and procoagulation. Because the ACE gene has been comprehensively conserved throughout evolution (including the great apes), taming a venom component has had clear advantages for science. Other venom derived drugs such as DEFIBRASE, AGGRASTAT, and INTEGRILIN, have also utilised the conserved evolution of the mammalian haemodynamic regulation system. However, Prialt is a chronic pain relief drug from venomous cone snails and it is unlikely these snails have evolved to reduce pain in animals. Some venomous cone snails of genus Conus prey on bony fish because they have evolved venom with potent calcium channel blocking peptides which immobilise their prey in seconds. This has enabled soft bodied invertebrates, which have to come out of their shell to feed, to attack the bony fish which often feed on snails. The venom rapidly blocks the neuromuscular signalling, leading to rapid paralysis and death in both fish and humans. It isn’t clear if the fish die before being eaten, as from the snails’ perspective it only has to stop moving to be eaten. A stretching hypothesis could conclude that blocking pain and movement in fish would aid stillness, but this link is only speculation at this point.
Another group of animals showing significant potential to deliver novel pain therapeutics are the theraphosid spiders, commonly and wrongly called tarantulas. These large hirsute spiders mostly prey on insects, even though some have collected names such as the bird eating spider. The term ‘bird eating’ seems to have stemmed from a copperplate print of 1705 by Joseph Mulder showing a large spider eating a hummingbird and the term stuck. Tarantula on the other hand stems from the European settlers who had a mystical dance called the tarantella which was performed to rid the victim of spider venom from a bite. The true tarantula is a small wolf spider from Europe and it’s now thought the actual bites that the dance was supposed to help were actually from a relative of the black widow. The name theraphosid is derived from the family theraphosidae of which the large spiders belong.
Despite the various names, the majority of theraphosid prey species are arthropods, mostly insects. Most arachnids employ neurotoxic methods to subdue their prey species and in this situation the most likely explanation as to why these venoms are so useful in pain therapeutics is down to divergent evolution. Inhibiting key Sodium channels in insects, such as the paralytic gene in Drosophila, is a very good way of preventing prey escaping and facilitating feeding. Mutations in the paralytic gene in flies are often lethal or produce significant locomotor defects as expected.
A mammalian orthologue of paralytic is the voltage gated sodium channel NaV1.7 (Gene SCN9A). In humans, loss of function mutations in SCN9A confer a congenital insensitivity to pain and thus, since this discovery, this target has received a lot of attention as a mechanism of treating severe pain without side effects. Several inhibitor cysteine knot peptides (ICK) from theraphosid venoms effectively block sodium channels including NaV1.7. Thus, it is clear to see venom that evolved to kill insects by blocking this channel has an innate potential as therapeutic agent in treating pain. Each venom also has several hundred different components, that of the Trinidad chevron spider, Psalmopoeus cambridgei contains many different ICK motif peptides that target Kv channels, ASIC1a and TrpV1. This venom also contains a potential new antimalarial peptide.
Venomous tool
There is no common venomous ancestor of spiders, centipedes and scorpions or between fish, snakes and mammals. Venom confers such an advantage to a species that there is a strong selection pressure to evolve it. Thus venom has evolved independently in all tetrapod clades, fishes and numerous arthropod lineages such that the diversity of potential tools in almost immeasurable. New venomous species are being discovered all the time and novel venoms being characterised include those from worms, crustaceans and primates. By employing modern drug discovery techniques, we are able to uncover the wide-ranging potential in venoms including tools for kinases, GPCR’s and ion channels without having to first wonder what the venoms evolved purpose is. This is because we don’t have all the answers to explain evolution completely but piece by piece we are getting there. Sometimes a novel therapeutic indication is discovered in a venom – that then informs us of the evolutionary benefit that component brings to the venomous animal.
Antibacterial compounds have been known in venoms since the 1970’s and antiviral activity since the 80’s but it is only through the recent discovery of the venom microbiome that this activity can be explained. There is an arms race in the venom gland between the host and the microbial colonisers and thus the antiviral activity in the venom compounds hints at a venom virome yet to be discovered.
It is true that animal venoms have not evolved to cure our diseases, and therefore we can dismiss any thoughts of anthropocentric evolution. But by remembering the targets of venom action have also evolved in humans and within the venom glands, we can open our eyes to a wonderful world of potential tools and therapies from venomous animals.
Steven Trim is Managing Director at Venomtech; www.venomtech.co.uk