Affordable carbon-loving materials for reduced industrial emissions
28 Jul 2020
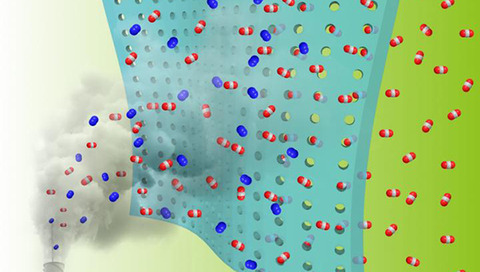
Researchers at the Department of Energy’s Oak Ridge National Laboratory and the University of Tennessee, Knoxville, are advancing gas membrane materials to expand practical technology options for reducing industrial carbon emissions.
Results published in Chem demonstrate a fabrication method for membrane materials that can overcome current bottlenecks in selectivity and permeability, key variables that drive carbon-capturing performance in real environments.
“Often there is a trade-off in how selective or how permeable you can make membranes that filter out carbon dioxide without allowing other gases to pass through. The ideal scenario is to create materials with high permeability and selectivity,” said Zhenzhen Yang of UT’s Department of Chemistry.
Gas membranes are a promising but still developing technology for reducing post-combustion or flue gas emissions produced by fossil-fueled industries.
The concept is simple: a thin, porous membrane acts as a filter for exhaust gas mixtures, selectively allowing carbon dioxide, or CO2, to flow through freely into a collector that is kept under reduced pressure, but preventing oxygen, nitrogen and other gases from tagging along.
Unlike existing chemical methods to capture CO2 from industrial processes, membranes are easy to install and can operate unattended for long periods with no additional steps or added energy costs. The catch is that new, cost-effective materials are needed to scale up the technology for commercial adoption.
“Gas membranes need pressure on one side and typically a vacuum on the other to maintain a free-flow environment, which is why materials’ selectivity and permeability are so important to developing the technology,” said Ilja Popovs of ORNL’s Chemical Sciences Division. “Underperforming materials require more energy to push gases through the system, so advanced materials are key to keeping energy costs low.”
No natural and only a few synthetic materials have exceeded what is called the Robeson upper limit, a known boundary that constrains how selective and permeable most materials can be before these rates start to drop.
Materials with sufficiently high selectivity and permeability for efficient gas separations are rare and often made from expensive starting materials whose production requires either long and tedious synthesis or costly transition metal catalysts.
“We set out to test a hypothesis that introducing fluorine atoms into membrane materials could improve carbon-capture and separation performance,” Yang said.
The element fluorine, used to make consumer products such as Teflon and toothpaste, offers carbon dioxide-philic properties that make it attractive for carbon-capture applications. It is also widely available, making it a relatively affordable option for low-cost fabrication methods. Research on fluorinated gas membranes has been limited because of fundamental challenges of incorporating fluorine into materials to realize its carbon-loving functionality.
“Our first step was to create a unique fluorine-based polymer using simple chemical methods and commercially available starting materials,” Yang said.
Next, researchers transformed, or carbonized, the material using heat to give it the porous structure and functionality needed for capturing CO2. The two-step process preserved the fluorinated groups and boosted CO2 selectivity in the final material, overcoming a fundamental hurdle encountered in other synthetic methods.
“The approach resulted in a carbon dioxide-philic material with high surface area and ultra-micropores that is stable in high-temperature operating conditions,” Yang said. “All of these factors make it a promising candidate for carbon-capture and separation membranes.”
The material’s novel design contributes to its exceptional performance, observed in high selectivity and permeability rates that exceed the Robeson upper limit, something only a handful of materials have accomplished.
“Our success was a material achievement that demonstrates feasible routes for leveraging fluorine in future membrane materials. Moreover, we achieved this goal using commercially available, inexpensive starting materials,” Popovs said.
The basic discovery expands the limited library of practical options for carbon-capture membranes and opens new directions for developing fluorinated membranes with other task-specific functionalities.
Researchers aim to next investigate the mechanism by which fluorinated membranes absorb and transport CO2, a fundamental step that will inform the design of better carbon-capture systems with materials purposely tailored to grab CO2 emissions.