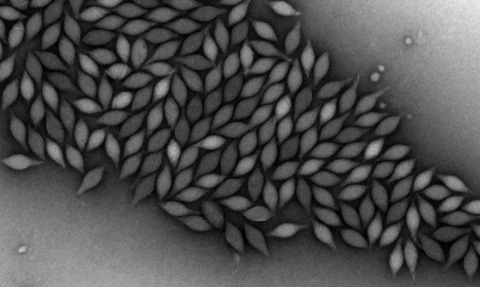
With diverse morphologies, our understanding of Archaeal viruses remains scant. Here, Prof Edward H Egelman discusses how his team applied advances in cryo-electron microscopy to uncover the atomic structure and unusual properties of lemon-shaped viruses.
More than 60 years ago it was realised by Crick and Watson that almost all viruses were either rod-like or spherical [1]. This is striking since the structure of these viruses is determined by the protein capsid that surrounds and protects the genome of the virus, and these protein structures have been independently ‘invented’ by different viruses over billions of years.
What Crick and Watson suggested in 1956 was that the rod-like viruses were helical, and the spherical viruses were icosahedral. In both cases, many copies of a single protein could exist in the capsid in equivalent environments.
Thus, the relatively small genome of a virus only needed to encode for a single capsid protein, reflective of the simplicity of viruses and a symmetry relating every subunit to every other subunit in the capsid.
However, for an icosahedral virus, there could only be 60 copies of a protein in such identical environments. It was, therefore, understood that large spherical viruses contained many more than 60 copies of the capsid protein. To account for this, in 1962 Caspar and Klug extended Crick and Watson’s argument about symmetry by introducing quasi-equivalence [2].
They explained how multiples of 60 subunits might exist in quasi-equivalent, rather than strictly equivalent, environments. Structural studies over many years have amply confirmed these arguments.
The revolution in cryo-EM
Alongside Bacteria and Eukaryota, Archaea represent the third domain of life. Viruses that infect Archaea are more diverse, less understood, and frequently deviate from the viral structure paradigm. For example, in some of the most extreme environments on earth, archaea that live in nearly boiling acid can be infected by viruses that look like spindles or lemons (Fig. 1).
In a newly published paper [3], my colleagues and I outlined research that determined the atomic structure of such a spindle-shaped virus. Our results show that this alternative structure still conforms to the principle of quasi-equivalence elaborated in 1962.
So, how was it possible to determine the atomic structure of such a virus? The answer involves a revolution in cryo-electron microscopy (cryo-EM) that began almost ten years ago [4].
Cryo-EM involves freezing samples so quickly that the surrounding water does not form crystalline ice, but rather a vitreous or amorphous glass. This prevents the damage that would normally occur for biological specimens when crystalline ice is formed.
Once frozen, samples are maintained at liquid nitrogen temperatures in the microscope for imaging. While the technology for doing this was developed in the 1980s, very few specimens were imaged at near-atomic resolution until several complementary technological advances became available.
The main factor in this revolution was the introduction of direct-electron detectors as cameras in electron microscopes. These replaced film or CCDs, which allowed for a tremendous improvement in the signal-to-noise ratio in cryo-EM images, and enabled much higher efficiency when actually recording electrons.
Combined with better microscopes and better software, these detectors made it possible to determine the atomic structures of a huge number of biological assemblies that could not be solved by other means.
Structural properties of a spindle-shaped virus
What does the atomic structure of a spindleshaped virus show? Remarkably, the protein found to form the capsid appears to be an integralmembrane protein, although there is no membrane in the virus! Proteins may be roughly divided into two categories: soluble ones, and integral-membrane ones. Integral-membrane proteins are characterised by being extremely hydrophobic, which causes them to reside partially or mainly within the highly hydrophobic interior of a membrane. The protein forms seven strands that pack together to form the viral shell (Fig. 2). [Figure 2: The seven strands forming the viral capsid, each shown in a different colour, see image below.]
The remarkable capsid can form a cylindrical tube after the genome is released. These properties show that it is the internal pressure of the genome that causes the structure to “balloon up” into a lemon shape in a similar way to a snake eating a rabbit.
It is the hydrophobic strands sliding past each other that allow these shape changes to take place, due to greasy surfaces that maintain the integrity of the capsid and keep out highly acidic water which might otherwise destroy the genome.
Implications of this discovery
The application of advanced near-atomic resolution cryo-EM microscopy gives us new insights into how viruses evolve. In this case how lemon-shaped viruses have evolved from rod-like ones to contain a larger genome.
There is also potential to make new structures in the laboratory that can exploit the unusual properties of hydrophobic subunits that assemble in the absence of a membrane. This could have implications for everything from new materials to new packaging for drug delivery or medical imaging. But like many fundamental discoveries in science, the path to technological applications can be a long one.
- Edward H. Egelman [pictured above] is the Harrison Distinguished Professor of Biochemistry and Molecular Genetics at the University of Virginia School of Medicine, virgina.edu
- The original paper was published in Cell. Search ‘Spindle-shaped archaeal viruses evolved from rodshaped ancestors to package a larger genome’ on cell.com
References:
- Crick FH & Watson JD (1956) Nature 177(4506):473-475.
- Caspar DL & Klug A (1962) Cold Spring Harb. Symp. Quant. Biol. 27:1-24.
- Wang F, Cvirkaite- Krupovic V, Vos M, Beltran LC, Kreutzberger MAB, Winter JM, Su Z, Liu J, Schouten S, Krupovic M, & Egelman EH (2022) Cell in press.
- Kuhlbrandt W (2014) Science 343(6178):1443-1444.