Turning drought into opportunity
30 Jun 2022
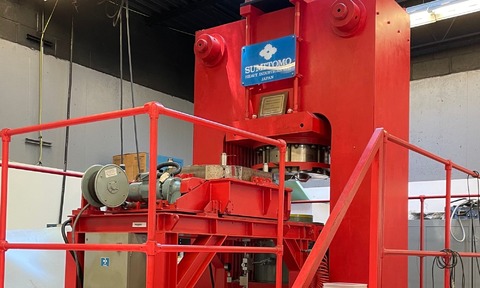
Mineral physics can help provide a solution to water shortage and its applications may not be limited only to our own world, suggests Sabrina Lautin.
On May 3, 2022, the LA Times published an article on the alarming drop in the water level of Lake Powell, a reservoir on the Colorado River of hugely consequential proportions. We may recognise this recent development as the calling card of climate change and its negative effects on the hydrosphere; however, what we have yet to acknowledge is the abundance of water beneath Earth’s surface. Within the mantle layer of the Earth, multiple oceans'–worth of water is contained in the crystalline structures of minerals, and on the cutting edge of the fight against climate-related catastrophe is a unique subdiscipline of geosciences: mineral physics.
Pioneered by scientists at Columbia’s Lamont Doherty Earth Observatory, and now carried out at institutions such as the State University of New York at Stony Brook, mineral physics characterises the study of Earth’s minerals at high pressure and temperature. With the goal of describing elastic behavior, sheer moduli, and deformation of the crystal lattice at extreme conditions, mineral physics encompasses the study of hydrous (water-containing) mantle minerals such as pyroxenes, garnets, silicates, and olivines.
Perhaps most integral to using the mantle as a water source is information on the true water content of each mineral found in high-pressure, high-temperature mantle conditions. For instance, we might observe 3.3 weight percent water content in hydrous wadsleyite, which then seems low in comparison with ‘phase D’, a high-pressure polymorph of hydrous magnesium silicate containing 10.1 weight percent water.
Some means by which to determine the water content of the mantle include tomography, or imaging of the mantle via low-frequency seismic wave propagation, as well as high-pressure laboratory analysis using a machine such as Stony Brook’s Sumitomo press (pictured).
With knowledge of a mineral’s elastic behavior and its abundance in the mantle, we can begin to make approximations regarding the exact quantity of water sequestered in the mantle
The seismology-driven approach reveals discontinuous wave speeds corresponding to pockets of different minerals in the mantle, while the recreation of deep Earth conditions in-situ can illuminate the unique properties of a hydrated crystalline structure subjected to pressures exceeding 1.4 million atmospheres; with knowledge of a mineral’s elastic behavior and its abundance in the mantle, we can begin to make approximations regarding the exact quantity of water sequestered in the mantle. There are, nevertheless, some nuances regarding mineral quantification that must be considered.
For instance, silicates, another class of hydrous minerals, exist as several polymorphs such as quartz and coesite (with the same chemical composition but different crystal structures). Consideration of these polymorphs lends further complexity to efforts directed at finding the mass of mantle-bound water. Mobility of a mineral within the actively deforming mantle can lead to the formation of what is called a metastable intermediate, where the pressure differential leads to a transitional form of the crystal lattice; for this reason, water may not be easily measured in mineral microstructures whose water contents are constantly in flux.
Olivine, a further example of transient mineral states, undergoes a process called serpentinization—upon interaction with seawater at a subduction zone, olivine becomes hydrolysed and forms either lizardite or chrysolite, and a highly H2-rich fluid byproduct. In short, it is possible to understand the exact transformations that occur, but this knowledge makes for an even more complex and algorithmic approach to engineering a mass balance of water in the mantle.
Mass-balance calculation of subterranean water could work on other planetary bodies, with implications for chondritic formation and accretion of astral bodies
One way in which we as scientists can harness an ocean’s worth of potential subsurface water is through high-pressure experimentation in a lab environment: perhaps the use of equipment such as the large, multi-anvil Sumitomo press at Stony Brook can characterise a continuous spectrum of the above-mentioned metastable hydrous phases of known mantle minerals. Olivine might be a good place to start, as it is widely available (relatively speaking) in polycrystalline form (multiple crystals fused together to form a sample of workable size).
The reason we need a continuous spectrum of mineral states is so that water can remain in the mineral structure of the sample long enough to reach room pressure and temperature conditions. The goal is extraction of water from the crystal lattice using lab techniques to create a continuous hydrous metamorphosis, and fortunately we already have most of the technology in place.
Ultimately, mineral physics as a discipline holds the solution to a problem as significant as drought, but ultimately the applications are out of this world. Mass-balance calculation of subterranean water could work on other planetary bodies, with implications for chondritic formation and accretion of astral bodies, as well as for astrobiology and the existence of water-dependent microorganisms. Indeed, water supports most life as we know it, and mineral physics may be the answer to fraught water politics and supply.
Sabrina Lautin is a PhD student at SUNY Stony Brook studying mineral physics, seismology, and rheology.
Sources:
https://www.sciencedirect.com/topics/earth-and-planetary-sciences/hydrous-mineral
https://link.springer.com/referenceworkentry/10.1007/978-94-007-6238-1_119
http://citeseerx.ist.psu.edu/viewdoc/download?doi=10.1.1.608.2854&rep=rep1&type=pdf
https://www.geochemsoc.org/files/4114/1270/3143/SP-6_283-296_Frost.pdf
https://agupubs.onlinelibrary.wiley.com/doi/full/10.1029/2018GC008090
https://agupubs.onlinelibrary.wiley.com/doi/10.1029/2020JB019375
https://earth.yale.edu/news/habitability-and-deep-mantle-water-circulation