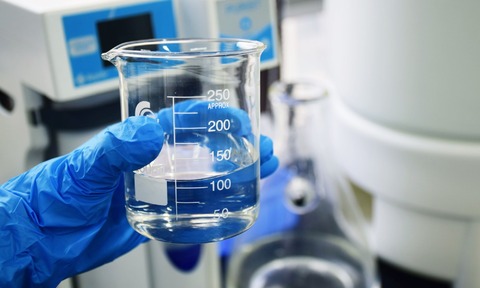
Time-consuming off-site manual testing is being supplanted in many areas by faster and greater capacity digital 24/7 field versions. James Flewellen details how one new collaboration could overhaul toxic material checks in water monitoring and other sectors.
Liquids and electronics are usually considered a potentially lethal combination. However, new technology currently in development could be a gamechanging blend between the two – providing a novel way to monitor for toxic chemicals and potential contamination in different fluids.
Thanks to funding and support from the Industrial Biotechnology Innovation Centre (IBioIC), the SIM card-sized tool is being produced and could transform routine health and safety testing across a range of industries. It could, for example, be applied to food and drink manufacturing, water monitoring, pharmaceutical development, agriculture and aquaculture to detect minute traces of chemicals.
At present, the best way of detecting chemical contamination in liquids is by manually taking samples and sending them away for expensive lab-based testing.
This is usually done infrequently, meaning problems caused by dynamically changing conditions, or transient events like excess oxygen, exposure to extreme conditions, or cross-contamination during production processes can go undetected for some time.
The new testing tool would allow businesses to check for foreign pollutants on-site or even remotely, providing real-time 24/7 readings, using a natural microorganism that causes a reaction, which is detected electronically, when certain chemicals are found. In contrast, labbased testing only captures a snapshot in time, giving a limited overview of the situation.
Fundamental questions, practical designs
The kernel of the idea was born from a research collaboration, funded by ONR Global, with experts in electronics from the University of California, Berkeley, and our own team of biophysicists at the University of Edinburgh. Initially, this was to explore the possible ways that electronics could be used to understand the energy generation of bacteria. To do that we wanted to measure ion flows on thousands of cells simultaneously, in contrast to the typical microscope-based research which only allows one to consider a single, or at best, a couple of cells at a time.
Rather than simply using the resulting technology for studying fundamental questions, we also wanted to use the technology as a standalone commercial method for detecting the presence of small molecules and other analytes in liquids.
From research tool to scalable product
The early proof of concept gave us confirmation that our bioelectronic interface technology could detect changes in the chemical makeup of a liquid, and this was based on lab-scale equipment and a prototype circuit board the size of a postcard.
The next stage was the process of refinement, analysing and recording electrical signals, and developing software to read and communicate the results. Alongside this came multiple discussions about the sectors which the tool would be beneficial for, and how to mak e it most suitable for their needs.
Considerations include waterproofing, the size of the device, how to best transmit the electrical output signals, the life cycle and battery requirements, and the user interface. We are still going through this process, working out what the end product might look like.
There are a number of ways the technology could be developed for different markets, and we are already in discussions with specialists from various sectors to explore the best next steps in terms of prototypes and testing.
The first could be an easy-to-use, low-cost device, similar to one of the later al flow testing kits we are all now very familiar with. Much like a Covid test, but likely smaller and much more rapid, this would return near-instant test results and quickly show whether certain toxins, contaminants or chemical nutrients are present in a liquid sample.
Secondly, it could be an automated fluid control device, suitable for use in industrial or environmental settings. For example, the device could be connected to pipelines and take regular readings to measure metabolic markers or monitor for evidence of crosscontamination. It could also sit within a floating case to take passive readings in different tanks, outdoor reservoirs or aquatic ecosystems.
Alternatively, it could be much more advanced, with integration in robotic devices such as remotely operated vehicles (ROVs) used by the offshore energy and subsea sectors. For particularly dangerous or hard-to-reach places such as underwater pipelines or wind turbine chambers, this could provide a much safer way to monitor for contamination or infrastructure degradation.
Next steps
One of the biggest advantages of the technology is that it could be applied broadly. However, this has subsequently made prioritising different testing scenarios and deciding how to progress the design challenging. For example, we could find the device being used by utility companies to check for minerals in drinking water reservoirs or in aquaculture to monitor for harmful algal blooms (HABs), but the same version of the device may not work in both settings.
The new testing tool would allow businesses to check for foreign pollutants on-site or even remotely, providing real-time 24/7 readings, using a natural microorganism that causes a reaction
Alongside the technology development process, we are also in the midst of spinning out as a new business from the University of Edinburgh and are keen to connect with the right partners and people to take that forward.
The biosensor device could be incredibly powerful for providing an early indication of potential contamination issues, allowing companies to intervene or take preventative measures. It is an exciting research breakthrough, and the ambition is to translate this into a commercial product which could be ready in the near future.
Dr James Flewellen is research associate and biosensor commercialisation team lead at the University of Edinburgh