SHERLOCK joins the hunt for rogue DNA and RNA
6 Jul 2017 by Evoluted New Media
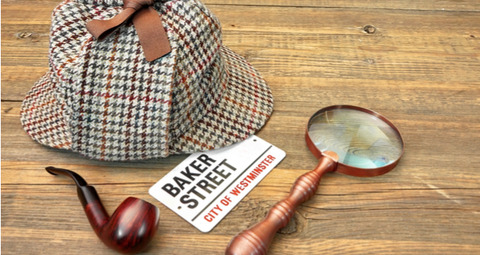
CRISPR – and the significant legal battles surrounding it – seem to be in the news more and more recently. Now researchers, with CRISPR as a building block, have found a way to detect mutated DNA in the bloodstream says Dermot Martin
CRISPR – and the significant legal battles surrounding it – seem to be in the news more and more recently. Now researchers, with CRISPR as a building block, have found a way to detect mutated DNA in the bloodstream says Dermot Martin
A new diagnostic tool for cancer with the potential to detect minuscule concentrations of mutant DNA in a patient’s blood has been created by the same team partly credited with developing the CRISPR gene editing technique.
The team, from The Broad Institute, Massachusetts, US, used a version of CRISPR to detect infinitesimal amounts of nucleic acids. It is hailed as a significant leap on the road to low-cost, accurate detection of cancer cells and to help researchers better understand how they develop over time.
The team describe the system as Specific High Sensitivity Enzymatic Reporter Unlocking, which abbreviates conveniently to SHERLOCK. Interestingly, it includes a reporter RNA strand that fluoresces when cleaved. Already the system is paving a route to detecting the Zika virus in blood and urine within hours of samples being taken. The team say the test is sensitive enough that it can distinguish between different strains of the Zika virus. Its apparent ability to rapidly read genetic information opens the door to detecting people at risk of heart disease from a simple saliva sample.
The system is being hailed as another triumph for CRISPR as it underpins how SHERLOCK works. This follows on from the work of Professor Jennifer Doudna and the University of California Berkeley, who last autumn published research hailing the use of CRISPR to detect nucleic acids.
Doudna’s team employed the Cas13a enzyme to observe collateral cleavage activity for RNA detection but it was hampered by the fact that it needed many millions of molecules and therefore lacked the sensitivity for clinical and research applications.
An April publication by Feng Zeng’s team is more than a nod to Doudna’s original paper offering further advances in disease diagnosis. It comes on the back of a legal impasse in the CRISPR patent dispute between to the Broad and Berkley which has attracted worldwide publicity.
The CRISPR debate
The high-profile dispute is not only about who takes the credit for development of CRISPR but also access to the pot of gold in potential royalties from licences and research spinoffs. What becomes clear from this work is that rivalry in the laboratory between the two sides is as fierce as it is in the courtroom.Professor Jennifer Doudna has already reacted to the latest paper by Zeng‘s team by pointing out that she had already reported the step involving the cleavage of a reporter RNA as part of her team’s efforts written up last year. This was in addition to her warnings scaling it to a point of care application will be challenging. Dr Doudna has pointed out that this new paper combines two previously reported methods.
She said: “First, there is a nucleic acid amplification step, to generate target RNAs for C2c2 (previous nomenclature for Cas13a) to detect. The MIT and other groups have published this approach in the past. Secondly, the amplified RNA is detected by C2c2, which can then cleave a reporter RNA to generate a fluorescent signal. This second step is what we published last year.
“The new method improves the limit of detection for C2c2 by pre-amplifying the nucleic acid target. The limitation is that it still requires the nucleic acid amplification steps. This could be challenging to scale into a point-of-care application,” she said
In a sense this tension between researchers could be driving CRISPR’s fortunes faster than would otherwise be the case –which is probably a good thing for the future of genetic research. Understanding CRISPR and the way it can alter the behaviour of genes is likely to help our understanding of antibiotic resistance simply because CRISPR itself emerged from the study of how bacteria fight back against disease that attack them.
Describing the new SHERLOCK system, Omar Abudayyeh co-author of the work told The Scientist: “While researching bacterial genomes for new CRISPR enzymes we discovered one we call Cas13a. This enzyme has unique properties we found – not only does it target RNA instead of DNA it also cleaves nearby RNA sequences.
“When it recognises its target it becomes crazy and will start chewing up anything else in the tube. We call this collateral activity (CA).” This CA is a key SHERLOCK characteristic which includes a reporter RNA which fluoresces when cleaved.
Johnathan Gootenberg, who also works in Feng Zeng’s lab was part of the same team. He said Cas13a initially had problems over sensitivity. “We found it wasn’t sensitive enough so we collaborated with James Collins, the biological engineering pioneer at MIT, to incorporate isothermal RNA amplification which Collins used to create a paper based Zika test.”
The team found that isothermal RNA made it possible to detect RNA and DNA molecules at attomolar (10-18 moles per litre) concentrations. According to researchers at Broad, the SHERLOCK system has the potential to become a paper based assay costing about 50p. They are currently planning a new start-up company once investment funding is confirmed.
What is CRISPR?
At the heart of the CRISPR phenomenon lies two molecules, which effectively work as a pair of scissors and a microscopic sat nav. The protein Cas9 is the ‘scissors’ which can chop up DNA. But precisely where it does so is directed by a short strand of ribonucleic acid (RNA).These two components evolved to help bacteria identify – and destroy – invading viruses’ DNA. Researchers worked out how to isolate and adapt these ‘tools’ to let them edit any gene with precision. First, the RNA ‘sat nav’ precisely matches up with a particular stretch of DNA. It brings the Cas9 molecule along with it, allowing the scissors to cut at that exact point in the DNA sequence.
CRISPR’s power as a research tool comes from being able to engineer bespoke versions of the RNA sat nav, allowing Cas9 to be directed to any gene a researcher wishes. But it’s what happens next that makes the CRISPR system a cellular version of the “find and replace” tool in a word processor. Once Cas9 has cut the DNA, the cell’s built-in repair machinery swings into action. Researchers can use this response to disrupt the gene that has been cut, essentially switching it off to see what happens.
Past articles by Dermot can be viewed here or here.
Author: Dermot Martin is a science journalist with a special interest in the life sciences and techniques for analytical chemistry.