The big squeeze
3 Mar 2015 by Evoluted New Media
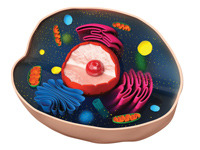
The physical properties of proteins, so vital for cellular function, are intimately linked to the cellular environment – a very crowded place indeed. But has this relationship been neglected in our understanding of their physicochemistry? Simon Ebbinghaus and David Gnutt think so, and they say they have a solution…
The physical properties of proteins, so vital for cellular function, are intimately linked to the cellular environment – a very crowded place indeed. But has this relationship been neglected in our understanding of their physicochemistry? Simon Ebbinghaus and David Gnutt think so, and they say they have a solution…
Proteins as well as other biomolecules are often analysed and characterised in highly diluted aqueous solutions. However, it is uncertain if such experimental approaches can be readily transferred to the densely packed cell environment.
For the first time, our method allows the measurement of the compression effects on proteins due to the lack of space in living cells with fluorescence microscopy. Depending on how crowded a certain environment in the cell is, a colour change can be detected. Our findings were reported in Angewandte Chemie and Angewandte Chemie International Edition.1
Biomolecules, e.g. proteins or nucleic acids, fulfil a wealth of different functions in a cell, such as catalysis or transmission of cellular signals. However, even small protein defects can have dramatic consequences: misfolding and aggregation of certain proteins are, for example, connected to severe diseases such as Alzheimer’s or Huntington’s disease. Often, such proteins are removed from their natural environment, the cell, and studied under controlled conditions in aqueous solution. Therefore, such studies often neglect the influence of the cellular environment, a densely packed matrix with macromolecules occupying up to 40% of the available volume.2 From a biophysical point of view, this raises the question whether studies in an aqueous solution can reflect the behaviour of a protein in a complex environment such as a cell.
A classical view describes the effects of such an environment as an effect of the “excluded volume”.3 Looking at a real life example nicely illustrates this theory: In a train full of people, everybody tries to minimise the contact to the other person by taking a compact posture. This concept can be translated to the microscopic world: due to the restricted space in a highly crowded environment, proteins favour a more compact state which naturally reflects the native fold of a protein. Therefore, looking solely on steric effects, proteins are more stable in highly occupied spaces. In order to probe this, artificial crowding substances were introduced. Indeed, a stabilisation of proteins can be observed when proteins are crowded with artificial crowding substances, e.g. Ficoll 70 or dextran.4 But can those substances realistically reflect the complex cellular environment?
In order to understand the effects of excluded volume in the cell, we introduced a new sensor based on a fluorescently tagged polyethylene glycol (PEG), a compound which is often, due to its inert and biocompatible nature, used as an artificial crowding substance itself. The PEG chain is terminally labelled with a green and a red dye. Once the chain is getting compressed, the closer proximity of the dyes allows a higher percentage of energy transfer (Förster Resonance Energy Transfer, FRET) from the green to the red dye (Figure 1). Thus, changes in the compression are indicated by a change of the detected colour.
[caption id="attachment_41942" align="aligncenter" width="450"] Figure 1: Illustration of the PEG sensor labelled with a green and a red dye at both ends in different concentrations of crowding compound (PEG, 10 kDa). Scale bar: 30 Å. Figure courtesy: Angewandte Chemie International Edition DOI: 10.1002/anie.201409847. [1][/caption]
It came as no surprise that under artificially crowded conditions in the test tube (e.g. with high concentrations of Ficoll 70, the copolymer of sucrose and epichlorohydrin) a strong compression of the sensor was observed. As this effect cannot be observed for sucrose, one can conclude that the compression is caused by the macromolecular nature of the crowding substances.
However, the situation inside a living cell is completely different. Although the cell is known to be highly crowded, introducing the sensor into cells by microinjection revealed similar conformations of the sensor in the cell as in diluted buffer. Therefore, the compression forces which are observed in artificially crowded systems are counteracted by forces that pull the sensor apart in the cell environment. Getting back to the macro-world analogy – one can imagine that people in a crowded train do not attempt to compact themselves anymore but instead allow contact with each other – a situation which might be true for football fans on their way to the stadium.
But what consequences does this have for proteins in a living cell? A general compression and thus stabilisation effect of the cellular environment, as predicted by classical theory, is too simple to explain the physical and chemical properties of the cell. This finding is reinforced by experiments from other groups showing that some proteins are stabilised while other proteins are destabilised by the cellular environment.5 The situation inside a cell gets even more complicated because the cellular crowding is not distributed homogenously. This can be demonstrated by the significant differences of sensor conformations between the cytosol and the nuclear compartment. Our results can explain the different unfolding times of phosphoglycerate kinase seen between both compartments.6
But what happens when you increase the concentration of macromolecules inside a cell? In order to investigate this question, we utilised an osmotic shock to simulate an even more crowded place. As one would expect, an increase in the macromolecular content of the cell readily leads to a compression of the sensor. Our results demonstrate that certain conditions can drastically change the cellular environment and its physical properties. Such changes could have strong influences on certain proteins, e.g. enhance its propensity to aggregate or to misfold. Alternatively, the cell could actively use such changes in the cellular crowding to regulate processes like the response to osmotic stress.7
Our study provides a new way to characterise the physicochemical properties of the cellular environment. We were able to point out that the cellular crowding effect can neither be described by a simple theory based on steric effects nor by experiments in artificial crowding substances. Nonspecific interactions, which can counteract or reinforce the steric excluded volume effects depending on the chemical nature of the protein, will have to be considered in cellular studies. We were further able to show that upon osmotic stress the cellular environment dramatically changes which could be linked to changes in the behaviour of proteins under such conditions. Henceforth, future studies will have to spatially and temporally resolve the properties of the cellular environment and correlate them with changes in the function of a protein.
References
1. a) D. Gnutt, M. Gao, O. Brylski, M. Heyden, S. Ebbinghaus, Angew. Chem. Int. Ed. 2014, DOI: 10.1002/anie.201409847; b) D. Gnutt, M. Gao, O. Brylski, M. Heyden, S. Ebbinghaus, Angew. Chem. 2014, DOI: 10.1002/ange.201409847.
2. a) S. Zimmerman, S. Trach, J. Mol. Biol. 1991, 222, 599-620; b) R. Ellis, A. Minton, Nature 2003, 425, 27-28.
3. A. Minton, Biopolymers 1981, 20, 2093–2120.
4. H.-X. Zhou, G. Rivas, A. Minton, Annu. Rev. Biophys. 2008, 37, 375-397.
5. a) K. S. Hingorani, L. M. Gierasch, Curr. Opin. Struct. Biol. 2014, 24C, 81-90; b) W. B. Monteith, G. J. Pielak, Proc. Natl. Acad. Sci. USA 2014, 111, 11335-11340; c) S. Ebbinghaus, A. Dhar, J. McDonald, M. Gruebele, Nat. Methods 2010, 7, 319-323.
6. A. Dhar, K. Girdhar, D. Singh, H. Gelman, S. Ebbinghaus, M. Gruebele, Biophys. J. 2011, 101, 421-430.
7. M. B. Burg, Cell. Physiol. Biochem. 2001, 10, 251-256.
Funding
The project was funded through the Rückkehrerprogramm run by the Ministry of Innovation, Science and Research in NRW, the Cluster of Excellence RESOLV (EXC 1069) run by the German Research Foundation as well as the International Graduate School of Neuroscience (IGSN).
Bibliophrapic record
Gnutt D, Gao M, Brylski O, Heyden M, Ebbinghaus S. Excluded-Volume Effects in Living Cells. Angew Chem Int Ed Engl. 2014 Dec 29. doi: 10.1002/anie.201409847. [Epub ahead of print]
The Authors:
David Gnutt, a PhD fellow of the International Graduate School of Neuroscience working in the laboratory of Junior Professor Dr Simon Ebbinghaus.
Simon Ebbinghaus, a Junior Professor at the Ruhr-University Bochum (Department of Physical Chemistry II). He worked as a Feodor Lynen Research Fellow with Martin Gruebele at the University of Illinois (Urbana-Champaign) from 2008 to 2010.
Contact: